Mysteries of the Hearing Brain: Can We Incorporate Real-World Signals in Clinical EEG Testing?
Because we use surface recordings (electrodes on the scalp) to measure electrical activity that arises from sources distant from the scalp, we typically average hundreds to thousands of responses to the same stimulus to obtain a response that is reliably above the noise floor. We are detecting extremely small electrical signals that range from 0.1 to 10µV depending on the source of electrical activity, and many averages are needed to cancel out random electrical activity. Auditory brainstem responses (ABRs) are smaller than cortical responses (e.g., late latency responses, LLRs), partly because they are generated at greater distances from the electrodes than cortical responses. For this reason, ABRs require many more stimulus repetitions than do LLRs. Envelope- or frequency-following responses (EFRs or FFRs) have multiple sources, including the brainstem, midbrain, and cortex, but they usually require >1000 stimulus repetitions to obtain an adequate signal-to-noise ratio (SNR) for examining features of the response in the time domain. Figure 1 displays LLRs for 10, 80, and 500 repetitions and FFRs for 500, 1000, and 2000 repetitions. Note that responses are observable for a relatively low number of repetitions, but the noise floor (the region prior to the onset of the stimulus) is quite high and this noise increases the overall amplitude of the response. It is advisable to try to reduce the noise floor, especially if you want to increase the reliability of the response or compare the response amplitude to normative values. For these reasons, relatively short stimuli (<200 ms) are usually recommended to ensure that testing times are clinically feasible.
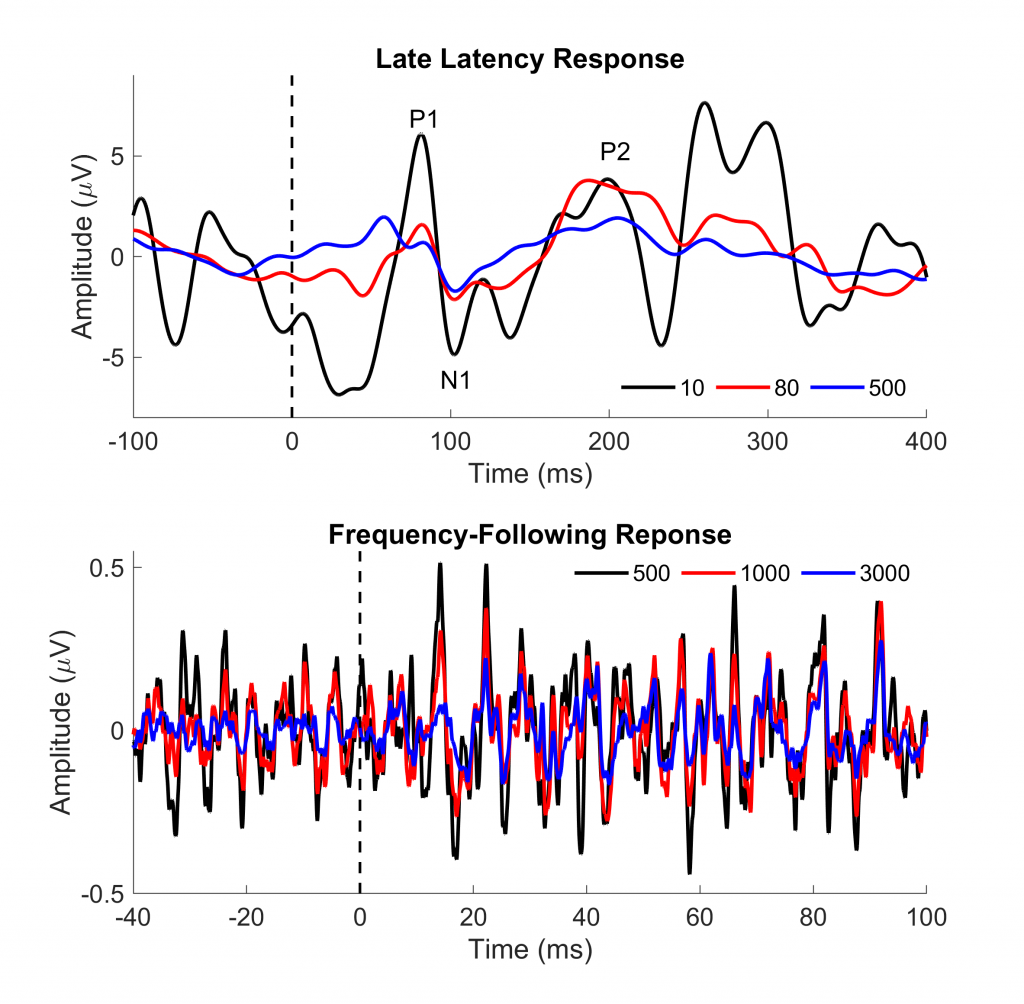
Figure 1. Top panel. The late latency response is displayed for 10, 30, and 500 averages. Note that the peaks (P1, N1, and P2) are easily visible with just 10 repetitions, but the noise level (region between -100 and 0 ms) is quite high. Bottom panel. The frequency-following response is displayed for 500, 1000, and 3000 averages. One can observe the periodicity of the peaks with just 500 repetitions. Again, the noise level prior to 0 ms is quite high, affecting reliability of the response.
Several labs are conducting innovative studies that incorporate speech signals into objective assessments of speech understanding. For example, Easwar and colleagues1 recorded EFRs to 300 stimulus repetitions of the speech token /susaʃi/, which had a duration of 2.05 seconds. The recording time for one condition was approximately 20 minutes, a duration that should be clinically feasible. The stimulus was chosen to encompass much of the speech frequency range, and a statistical analysis was used to determine if a response was detectable above the noise floor at different frequencies. The study concluded that this protocol could be used as an objective hearing aid outcome measure, especially for infants, children, or other individuals who are not able to provide behavioral responses.
Adults are usually able to provide the kind of feedback needed to determine if a speech signal is audible, but they often have difficulty verbalizing problems related to speech quality. The audiogram is not a good predictor of speech understanding in noise, and it may be useful to incorporate objective measures of speech understanding when evaluating hearing ability in real-world environments. Methods have been developed to assess the brain’s ability to follow speech samples of relatively long direction (e.g., one minute) that are presented with just a few repetitions. This type of measurement could help the clinician assess how well a patient would be able to follow a conversation while wearing hearing aids. The envelope of the speech signal can be reconstructed from the neural response, and then this reconstructed envelope is correlated with the actual envelope of the speech signal to obtain a measure of response fidelity.2 In Figure 2, one can see that the reconstructed envelope generally follows the speech envelope in a recording from a young adult. Recent studies have demonstrated that reconstruction accuracy relates to speech understanding in both younger3 and older4 listeners.
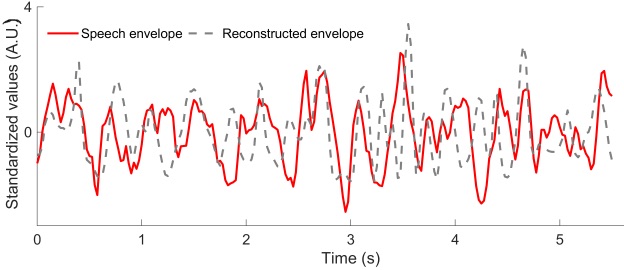
Figure 2. Waveforms from the original speech envelope (red) and the reconstructed neural envelope (red) are overlaid. One can see that the peaks of the speech envelope align well with the peaks of the neural response in this young listener.
Although this type of recording seems to have promise for future clinical applications, it is not yet clinically feasible. Currently, a 64-channel recording is necessary to acquire a response that is sufficiently above the noise floor. So, while the actual recording might be accomplished in ten minutes or less, application of the 64-channel cap generally requires at least 30 minutes. And, current clinical EEG recording systems do not have the capability of 64-channel recording. It is hoped that analysis methods can be developed to extract the neural signal with fewer channels. The ability to record responses to sentence-length or longer speech stimuli would greatly expand our ability to objectively assess real-world hearing ability.
References
- Easwar V, et al., Evaluation of speech-evoked envelope following responses as an objective aided outcome measure: Effect of stimulus level, bandwidth, and amplification in adults with hearing loss. Ear Hear 2015;36(6):635–52.
- Presacco, A., J.Z. Simon, and S. Anderson, Effect of informational content of noise on speech representation in the aging midbrain and cortex. J Neurophysiol 2016;116(5):2356–67.
- Vanthornhout J, et al. Speech intelligibility predicted from neural entrainment of the speech envelope. J Assoc Res Otolaryngol 2018; 19(2):181–91.
- Decruy L, Vanthornhout J and Francart T. Evidence for enhanced neural tracking of the speech envelope underlying age-related speech-in-noise difficulties. J Neurophysiol 2019.