Risk Factors Associated With Environmental Pressure Changes on Tympanic Membrane Rupture and Ear Damage
A first concern when presenting pressure into the ear canal that involves the tympanic membrane relates to risk – will it be uncomfortable, could it rupture the tympanic membrane (eardrum), could it cause other damage to the external auditory canal, or have other side effects? This rational question must be answered about any product that results in pressure changes in the ear canal.
The primary concern of pressure application in the ear canal relates to the tympanic membrane (TM). Pressure in the ear canal, especially if it is forceful (blast) or presented as a sound source, impacts the tympanic membrane. The human ear can tolerate a wide range of pressures, but when they become too great (overpressure), they can cause damage to the eardrum. This is most often in the form of a ruptured eardrum.
There are many causes of eardrum rupture. They include but are not limited to pressures from middle ear problems, blasts, sudden or constant loud noises, physical blows to the ear, and pressure from deep-water swimming. A typical overpressure required to guarantee a rupture to an eardrum would be about 100,000 pascals (100 kPa, or 14.5 psi), but rupture could occur at lower pressure levels with these data shown later.
Brief Anatomical Description of the Tympanic Membrane (Eardrum) and Eustachian Tube
A brief anatomical description of the tympanic membrane is warranted to describe why an eardrum can rupture. The eardrum (tympanic membrane) consists of three thin layers, with the outer and inner layers continuous with the tissues of the outer ear canal and the middle ear, respectively. The middle layer is fibrous, containing both radial and concentric features. Thus, the tympanic membrane provides some protection to the middle and inner ears and receives sound vibrations from the outer ear and transmits them to the middle ear.
The middle ear operates normally when it is filled with air at atmospheric pressure.
Biologically, the Eustachian tube must be considered in discussions of eardrum rupture. The Eustachian tube functions to equalize pressure on both sides of the TM. This tube normally opens and closes periodically, thereby ensuring that the static pressure in the middle ear will remain the same as atmospheric pressure. In addition, the Eustachian tube has elastic properties and extends from the nasopharynx (back of the throat) to the middle ear cavity. Normally closed, it dilates during yawning, swallowing, shouting, chewing.
Eardrum Rupture
Because the TM is so thin, it can be ruptured or punctured, which can occur from the outside by excessive pressure occurring so rapidly that the Eustachian tube cannot manage pressure equalization.
The eardrum can rupture in different ways: water pressure, differential pressure, and blast waves. Differential pressure is defined as the difference between two points. This represents the pressure on both sides of the eardrum – the atmospheric and middle ear. While differential pressure is of interest, essentially all reporting on eardrum rupture relates to blast waves.
Caveat: Because eardrum rupture tests cannot be performed on humans, the numbers for eardrum rupture have been almost theoretical and are more available for animals.2 The numbers used to predict rupture have been discovered by environmental, occupational, or clinical experiences rather than from experiments. These have been based on blast waves rather than on gradual changes (positive and negative), where the middle ear has had an opportunity to equalize.
Pressure
Pressure is the force per unit area applied on a surface in the perpendicular direction. The pressure equation is:
P = F/A P = pressure
F = force
A = area
Pressure is measured in pascals (Pa). One pascal is equal to a newton per square meter [Pa = N/m2].
To relate this to something understandable, this translates to 0.00014 psi (pounds per square inch), a minimal pressure – too small for reasonable use. For this reason, the pascal is used, where 100 pascals equals 0.01 psi – still a very low value, but something that one can somewhat get a handle on, primarily if related to tire pressures. And, because numbers related to the pressures in pascals become so large, it is convenient to relate the pressures in kPa, where 100 pascals is equal to 0.1 kPa.3
How Much Pressure to Rupture The Eardrum?
The tympanic membrane can tolerate reasonably high pressures but does reach a level where the amount of pressure can no longer be tolerated. As a result, the eardrum may rupture at sudden overpressures (blast). In a blast, an almost instantaneous wave of overpressure travels at a velocity greater than that of sound, followed by a region of gas flow.4 However, eardrum rupture can also be caused by pressure changes in atmospheric pressure (diving, travel in an aircraft) or by a rapid change in ear pressure in a normal atmosphere.
Blast Studies
Results of eardrum rupture (100%) from blasts is shown in Figure 1 with pressure referenced in psi (pounds per square inch). The pressure levels are fairly consistent, being between 14.5 and 14.9 psi.2,5,6–9 The orange column is used as a general psi pressure for 100 % eardrum rupture.10 That level is set at 14.5 psi.
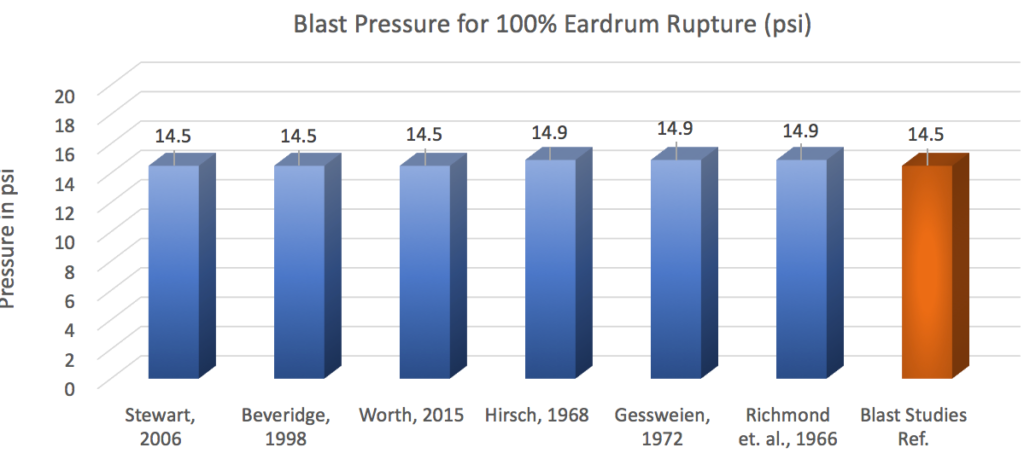
The data in Figure 2 represents the best estimates of eardrum rupture to blast pressure. Unlike the blast pressure for 100% eardrum rupture, considerable variation exists. Almost half (42%) show the pressure for 50% rupture at 5.1 psi, but the group, as a whole, shows a range from 2.2 to 12.2 psi.5,6,11–14 The orange column is used as a general psi pressure reference for 50% eardrum rupture.10 That level is set at 5.1 psi. The fact that five publications listed 5.1 psi as the 50% rupture pressure suggests that their 35 kPa (5.1 psi) reported data might be more of a consensus generalization.
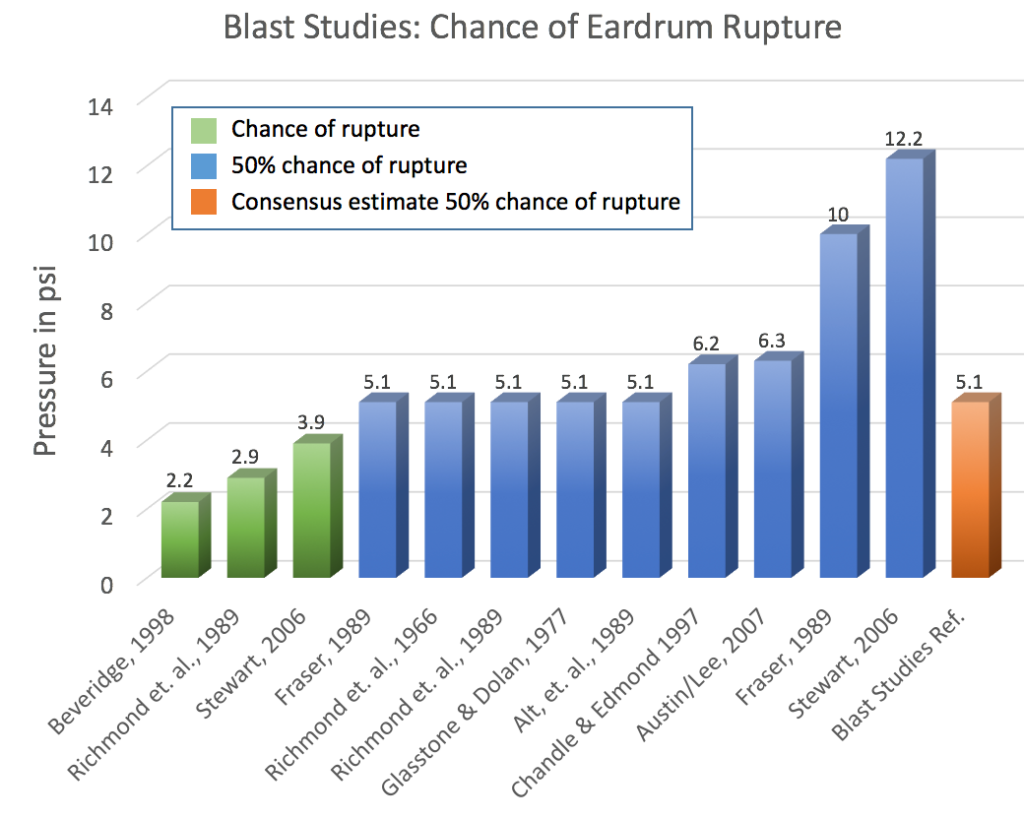
Blast Pressures For “Normal” Eardrum Rupture vs. Non-Blast Rupture Pressures
As mentioned previously, a significant problem with determining the pressure levels for eardrum rupture in a normal population is that rupture tests cannot be performed on humans. As a result, eardrum rupture in humans has been almost theoretical and uses environmental, occupational, or clinical experiences rather than human experimental data. Additionally, blast eardrum rupture data is not based on gradual changes (positive and negative) where the middle ear has had an opportunity to equalize. Under equalization, data suggest the ability to tolerate higher pressures.
Blast Trauma
Medical problems resulting from exposure to a shock wave can be divided into those that result from the static component and those that result from the dynamic component. Injuries resulting from blast waves are caused by exposure to high pressures with very short rise times and can rupture the eardrum at about 5 psi.15
That blast trauma operates differently than a gradually-rising pressure was recognized early on and is expressed as follows by Hirsch8.
“The vulnerability of an eardrum depends not only on the parameters of the blast wave at the site of the tympanic membrane (e.g., peak overpressure, rise time, and duration of the pressure impulse), but it also varies with the age of the eardrum, and depends on whether it has been subjected to previous trauma or disease.”
Hirsch continues,
“Based upon previous quantitative studies, it can probably be safely said that the pressure for damage to middle ear structures is about 5 psi (0.34 bar) and that overpressure near 15 psi (1.03 bar) will cause the rupture of 50% of the eardrums exposed to it.”
Slow-Rising Pressure
However, some information can be gleaned on data from human cadaver specimens on the breaking strength of a slowly rising positive air pressure, as measured by Zalewski and reported by Wever and Lawrence16 (Figure 3). He pumped air into sealed ear canals to prevent air from escaping. An average braking pressure of 23.2 psi (111 ears) was reported for normal-appearing eardrums, with a range from 5.8 to 43.5 psi. Abnormally thin eardrums (n=12) broke at an average of 7.5 psi, and scarred eardrums (n=12) broke at an average of 4.4 psi. Zalewski also reported that eardrums from the first decade of life broke at 33.1 psi, and older age groups at 20.0 psi.17 Overall, he reported that 11% broke at less than 14.7 psi, 66% broke between 14.7 and 29.4 psi, and 23% broke at greater than 29.5 psi.
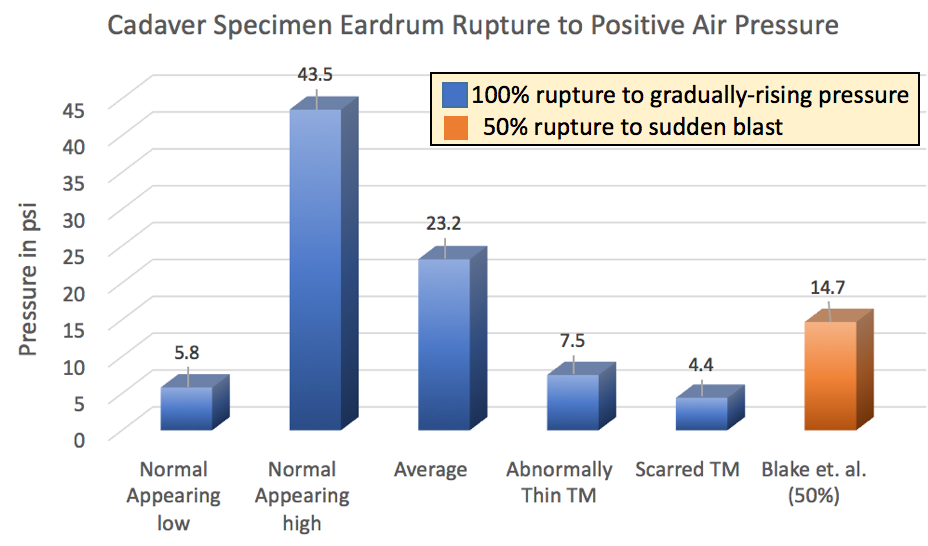
Another study on eardrum rupture on cadavers by Blake et al. 18 used a shock wave to burst the eardrum, rather than the slowly-rising overpressure used by Zalewski. They found that the pressure required to rupture the eardrum 50% of the time was at 14.7 psi (orange column in Figure 3).
Age
That age is a condition related to eardrum rupture is supported by Glasstone and Dolan12 in reviewing eardrum rupture following nuclear blasts. Individuals over 20 years of age showed eardrum rupture at 15 to 20 psi, while individuals under 20 showed eardrum ruptures at a higher pressure, from 30 to 35 psi.
Ear Canal Sound Pressures Representative in the Human Ear
A first concern when presenting pressure into the ear canal that involves the tympanic membrane relates to risk – will it be uncomfortable, will it rupture the tympanic membrane, will it cause other damage to the external auditory canal or other side effects?
Concerning safety, the most significant risks associated with pressure changes in the ear are pressure-induced ear discomfort or pain, and more seriously, damage to the tympanic membrane (e.g., tympanic membrane rupture).
Such devices used in otology and audiology employ pressures ranging from +0.9 psi to -1.2 psi.19 As outlined in a paper published by the American Speech-Language-Hearing Association (ASHA) Working Group on Aural Acoustic-Immittance Measurements about pneumatic systems, the paper explains that Type 1 and 2 instruments must be able to make pressure changes between +200 and -600 daPa. It also states that the instruments should not produce more than 600 daPa or less than -800 daPa (e.g., +0.8 psi and −1.2 psi) as they might cause damage to otherwise weakened tympanic membranes.
The data in Figure 4 shows the pressures (in psi) associated with various tympanic membrane activities.
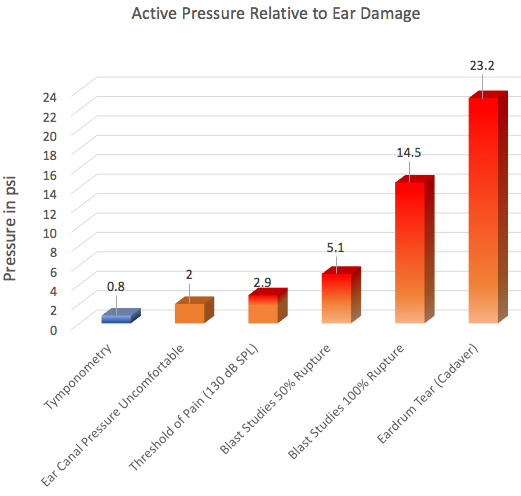
Ear canal uncomfortable levels occur around 2 psi (around 110 to 120 dB SPL), and the threshold of pain to pressure stimulation of the tympanic membrane is considered to be around 130 dB SPL (sound pressure level), or 2.9 psi.23-25 This level does not ordinarily rupture eardrums.
Concerning the potential for tympanic membrane rupture by overpressure, studies suggest that the minimum overpressure value required to produce minor or moderate tympanic membrane rupture is around 2.9-5.1 psi when the pressure is created as a result of a sudden and prolonged blast. If the ear has an opportunity to equalize the pressure on both sides of the eardrum from a gradually rising pressure (Eustachian tube function), data presented has shown that a higher pressure is required to rupture the eardrum.
Device Pressure Against External Ear Canal Wall
An element of risk occurs when a clinician performs an invasive procedure, such as placing an object into the ear canal. The trauma to the tympanic membrane has been addressed in the previous discussion and is considered a non-issue.
Ear Canal Wall
A subsequent question relates to the pressure regulating device and its impact on the ear canal wall during inflation and deflation.
Medical Inflation. Air inflation otoscopy (pneumatic-otoscope) is very useful to evaluate middle ear disease. This procedure is used to assess the mobility of the tympanic membrane by applying non-calibrated positive and negative pressures with the rubber squeeze bulb into the external auditory meatus (canal).
3D Imaging. A device that produces pressure in the ear canal by inflation and can be used for comparison purposes is the Lantos Technologies 3D ear scanning system. In this commonly-used system for ear impressions, a soft membrane at the end of the hand-held scanner has a visible otoscope tip guided deep into the ear canal by a video feed. Once inserted, the membrane is filled with a water-based optical dye, expanding until it conforms to the patient’s unique ear canal. Using a dual wave-length algorithm, the scanner takes thousands of 2D digital images as it exits the ear and stitches them together in real-time to create a highly accurate 3D image of the ear.
The scanner can even measure canal wall elasticity by varying the pressure inside the membrane and recording the results. This is done by allowing the inflatable membrane to stretch to greater degrees, such as 100% beyond its original dimension, 150%, 200%, and so forth26.
Although no data has been found as to the pressure in psi generated by the higher inflation options, it can be assumed that pressures would not be accepted if greater than discomfort. This level is assumed to be less than 2 psi. Lantos states that discomfort is similar to experiencing mild changes in ear pressure.27 With its optional increased high pressure options, the Lantos device is considered safe by the FDA (Federal Food and Drug Administration), which provided market clearance without restrictions in 2013.28
Earpiece Seal
Related to the pressure against the canal wall is the comfort of an earpiece, having an external complaint surface configured to sealably engage an external ear canal and serve as a barrier between the external ear canal pressure and ambient pressure.
Earpieces are used for hearing aids, ear protection, swim plugs, musician in-ear monitors, communication and 2-way radio, sleeper plugs, headphones, pilot earphones, and other devices directed toward the ear.
Earpieces are often intended to act as barriers to seal the ear canal from ambient pressure and are not new in the hearing and associated industry.29 Hearing aids, and later earplugs to reduce ambient sounds from entering the ear canal, have been common-use items starting in 192630, including soft non-custom earpieces by Lieber since 1933.31 Lieber’s contribution related to a patent intended to improve earpieces for hearing aids using a flexible material (such as soft rubber) that may yield somewhat and thereby adapt itself to the individual ear. This concept continues as an option to this day, not only for hearing aids and earplugs but also for earbuds and other hearable devices. The primary materials used to hold/contain devices in the ear include acrylic/lucite, vinyl (PVC – polyvinyl chloride), polyethylene, and silicone. Regardless of the material used, most human ears appear to be softer than earmold materials. If so, the ear tissue must conform to the earmold, not the earmold to the ear.32
Summary
A primary concern with generating pressures in the ear canal relates to potential tympanic membrane damage. This paper presented data relative to the psi levels leading to eardrum rupture from blasts, slowly-rising pressures, controlled intentional rupture on cadevers, comparison to commonly-used devices that use encapsulated ear pressure in the ear canal for diagnostic purposes, and daily-use devices that fit into the ear canal that have similar sealing properties.
Note: In this paper, pressure levels were converted from other pressure measurements (Pa, daPa, kPa, ATM, dynes/cm2, mm water, bar, and dB SPL to psi) for ease of reading and pressure comparisons.
References
- External ear pressure regulating system. (2015). U.S. Patent No. US 9,196,277 B2.
- Richmond, DR, Damon EG, Fletcher ER, et al. The relationship between selected blast-wave parameters and the response of mammals exposed to air blast. Lovelace Foundation for Medical Education & Research, Albuquerque, NM. 1966. Contract No: FA-49-146-XZ-372.
- Staab WJ. Eardrum rupture – at what pressure? Hearing Health and Technology Matters, January 15; 2012. Available at: http://hearinghealthmatters.org/waynesworld/2012/eardrum-rupture-at-what-pressure/.
- Fisher T. Blast injury of the ear. Ministry of Defence, Edinburgh, Scottland, September 2008.
- Stewart C. Blast Injuries: Preparing for the Inevitable. Emergency Medical Practice 2006;8(4).
- Beveridge A. Forensic Investigation of Explosions. Boca Raton. CRC Press; 1998: 1.
- Worth E. Ear pathology in SCUBA diving or freediving. Hearing Health and Technology Matters; 2016. Available at: http://hearinghealthmatters.org/waynesworld/2016/ear-pathology-scuba-diving-freediving/
- Hirsch FG. Effects of overpressure on the ear – a review. Technical Progress Report on Contract No. FA-49-146-XZ-372, Supported by the Defense Atomic Support Agency of the Department of Defense, Lovelace Foundation for Medical Education and Research, Albuquerque, NM; 1966.
- Gesswein J and Corrao P. The Position of Eardrum Rupture and Hearing Loss in the Scale of Injuries From Nuclear Blast. Bethesda: Naval Ship research and Development Center, 1972: 36.
- Richmond D, Yelverton J, Fletcher E, Philips Y. Physical correlates of eardrum rupture. Annals of Otology, Rhinology, and Laryngology 1989;109: 35-41.
- Fraser TM. The Worker at Work: A Textbook Concerned with Men and Women in the Workplace. CRC Press: 1989;323.
- Glasstone S and Dolan PJ. The Effects of Nuclar Weapons, Third edition. United States Department of Defense and the Energy Research and Development Administration; 1977;552.
- Alt L, Forcino D, and Walker R. Medical Consequences of Nuclear Warfare. Office of the Surgeon General Department of the Army, United States of America; 1989: 7.
- Chandler DW and Edmond CV. Effects of blast overpressure on the ear: case reports. J Am Acad Audiol 1997;8:81-88.
- Textbook of Military Medicine, Part I, Warfare, Weaponry, and the Casualty, Vol. 2, Medical Consequences of Nuclear Warfare, Series on Combat Casualty Care, Office of the Surgeon General, Department of the Army, United States of America, Alt, L.A., et.al (13 total), page 7, Falls Church, VA. 1989.
- Wever EG and Lawrence, M. Physiological Acoustics, Princeton; 1954:416.
- Zalewski T. Experimentelle untersuhungen ueber die resistheitsfaehigkeit des trommelfells. Zeitshr. F. Ohren. 52:109-128, 1906.
- Blake PM, Dougles JWB, Krohn PL, and Zuckerman S. Rupture of eardrums by blast. Medical Research Council Report B.P.C. 43/179/W.S. 21, Oxford, England; nd.
- American Speech-Language-Hearing Association (“ASHA”). Tympanometry [Relevant Paper]; 1988. Available from www.asha.org/policy/RP1988-00027/
- Jerger JF. Clinical experience with impedance audiometry. Arch. Otolaryngol 1970;92:311-324.
- ANSI, American National Standards Institute. American national standard specifications for instuments to measure aural acoustic impedance and admittance (aural acoustic immittance). New York: American National Standards Institute, ANSI S3.39-1987; 1987.
- ASHA, American Speech-Language-Hearing Association. Guidelines for scfeening for hearing impairment and middle ear disorders. ASHA 1990:32(Suppl.2):17-24.
- Franks JR, Stephenson MR, Merry, Carol J, eds. Preventing Occupational Hearing Loss - A Practical Guide (PDF). National Institute for Occupational Safety and Health 1996;88. Retrieved 2009-07-15. Available at: http://www.cdc.gov/niosh/docs/96-110/pdfs/96-110.pdf The threshold for pain is between 120 and 140 dB SPL.
- Newman EB. (1972-01-01). Speech and Hearing. American Institute of Physics handbook. New York: McGraw-Hill. pp. 3–155. ISBN 007001485X. The upper limit for a tolerable intensity of sound rises substantially with increasing habituation. Moreover, a variety of subjective effects are reported, such as discomfort, tickle, pressure, and pain, each at a slightly different level. As a simple engineering estimate it can be said that naive listeners reach a limit at about 125 dB SPL and experienced listeners at 135 to 140 dB.”
- Nave Carl R. Threshold of Pain. HyperPhysics. SciLinks. Retrieved 2009-06-16. “A nominal figure for the threshold of pain is 130 decibels ... Some sources quote 120 dB as the pain threshold.” Available at: http://hyperphysics.phy-astr.gsu.edu/Hbase/sound/intens.html
- Inflatable membrane for fluorescent imaging and improvements in dye materials. US Patent No. 20140272221 A1. Filed, March 14, 2014.
- Hsu J. 3D ear scanning enables custom-fit headphones. Innovation, 1/9/2013.
- Lantos Technologies receives FDA Clearance for its 3D ear scanning system, 3/18/2013. Available at: http://www.biospace.com/News/lantos-technologies-receives-fda-clearance-for-its/290736.
- Staab WJ. Ear impression history, Hearing Health and Technology Matters, August, 2016. Available at: http://hearinghealthmatters.org/waynesworld/2016/ear-impression-history/.
- Halsey AF. U.S. Patent No. 1,601,063, Acoustic Device; 1926.
- Lieber H. Earpiece for ear phones, US Patent No. 1,893,474. Filed, May 27, 1931; granted Jan. 3, 1933.
- Maye V. Field return analysis by account. Internal study., Canada: Starkey Labs; 1997.