Mysteries of the Hearing Brain – Music and the Hearing Brain
While pursuing my PhD with Dr. Nina Kraus at Northwestern University, many of my fellow students were investigating the effects of music training on the neural representation and perception of speech signals. When I told my husband about this line of research, he was quite skeptical, perhaps because as a violin maker he has worked with many musicians who believe that they are pretty awesome at everything! Even though he works with instruments, he is not a musician himself. I eventually persuaded my husband to participate in one of the experiments. Not surprisingly, his response looked like that of a musician’s, and when I pointed this fact out to him, he became a believer!
When I say that his response "looked like that of a musician's," I am referring to a higher representation of harmonics that is one of the key signatures of the musician's frequency-following response (FFR). As a violin maker, my husband needs excellent timbre (harmonic) perception, so it makes sense that his neural response would be rich in harmonics. Figure 1 displays an individual non-musician's response and a musician's response in the time and frequency domains. In the time domain, one can see that the peaks of the non-musician's response are higher in amplitude than the peaks in the musician’s response. On closer inspection, however, one can see that the in-between peaks are higher in the musicians, especially in the region corresponding to the stimulus vowel (60-120 ms). These in-between peaks correspond to the higher frequency harmonic content of the response. Also, the magnitude of the fundamental frequency (100 Hz) is lower while the magnitude of the second harmonic is higher in the musician compared to the nonmusician. This finding has been observed in children, young adult, and older adults.1
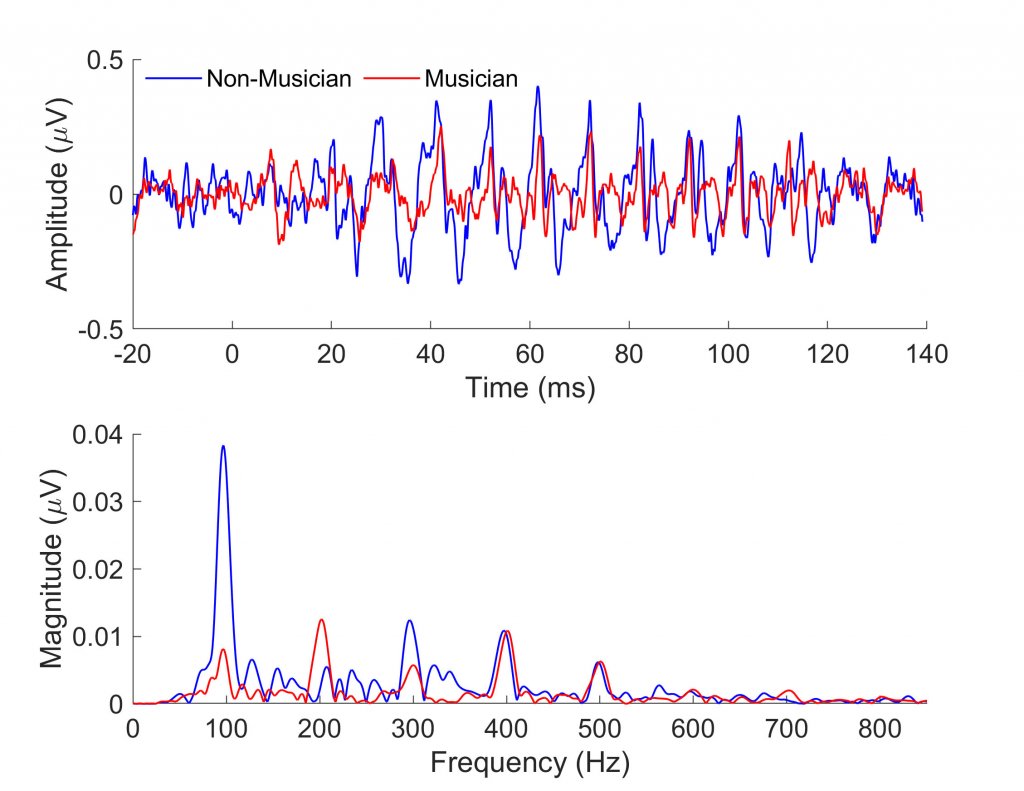
This neural enhancement in the higher frequencies has been interpreted to reflect neuroplasticity induced by music training, but this interpretation is controversial. Inclusion criteria in many music training studies specify that the musicians must have begun music training on or before the age of seven.2,3 Children that start music lessons at such a young age are likely to come from homes with a relatively high socioeconomic status (SES), in which they may be exposed to a variety of stimulating and enriching experiences that could lead to more robust neural function.4 Another confounding factor in these music studies is that innate musical aptitude may motivate a child’s desire to pursue music training, and it may play a role in the musician's neural enhancement. Music listening ability can be objectively evaluated using the Profile of Music Perception Skills (PROMS), which tests perception of melody, tuning, accent, and tempo perception.5 Mankel and Bidelman6 used the PROMS to determine the extent to which music listening modulates auditory neural processing in two groups of young adults who were divided into high- and low-scoring PROMS groups. Both groups had minimal formal music training (< 3 years) and were matched on age, SES, and years of formal education. Mankel and Bidelman found that the high PROMS group had a higher representation of both the fundamental frequency and the harmonics in FFRs to the speech syllable /a/ presented in multi-talker babble. These results suggest that innate music ability plays a role in musicians’ enhancement of auditory neural responses to auditory stimuli, and this natural aptitude may be further developed or specialized with formal music training.
Strait et al.7 demonstrated how natural music ability may be specialized by training on a specific musical instrument. They recorded FFRs in musicians who had been trained to play the piano and in musicians who had been trained to play another instrument; the groups were matched in years and onset of music training. The stimuli included three music sounds, piano, bassoon, and tuba, which differed only in timbre (harmonics). The pianists' neural responses to the piano note had higher morphology than the non-pianists’ responses, but there were no group differences in responses to the sounds produced by the bassoon or tuba (only one participant had played the bassoon). The authors concluded that this study provided evidence for experience-dependent plasticity beyond innate ability. This experience-dependent specialization of neural responses in musicians has also been demonstrated in a series of studies that recorded cortical event-related potentials in musicians with different music training backgrounds.8 In their studies, the investigators found that conductors showed better spatial tuning (mismatch negativity response [MMN] to a deviant stimulus coming from an unattended direction) compared to the MMN recorded in pianists. Further, they found that drummers’ MMN responses were more tuned to the “odd” beat in a drum sequence compared to the MMN recorded in woodwind players.
Audiologists are most interested in interventions that lead to better speech understanding. However, the evidence for the benefits of music training on speech-in-noise (SIN) performance has been mixed. A series of studies in Nina Kraus’ lab showed better SIN performance in musicians compared to nonmusicians across age groups.9–11 Other studies, however, have shown equivalent SIN performance between young adult musicians and nonmusicians.12,13 One possible reason for the discrepancy may be a difference in the age that musicians started training. For example, the mean age of onset was 4.9, 5.9, and 8.2 in Parbery-Clark et al.,10 Ruggles et al.,12 and Escobar et al.,13 respectively. Perhaps the age of onset is a critical factor in the extent to which music training can affect SIN performance. On the other hand, SES is likely to be a factor in families who can afford to provide music lessons for children who are under five years, and factors related to SES may also influence SIN performance. A few longitudinal studies have demonstrated improvement in children’s SIN performance with music training over time.14,15 More studies like this are needed to determine whether music is a viable intervention, especially in individuals with hearing loss.
References
- Strait DL and Kraus N. Biological impact of auditory expertise across the life span: musicians as a model of auditory learning. Hear Res 2014;308:109–21,
- Strait DL, et al. Musical experience and neural efficiency: effects of training on subcortical processing of vocal expressions of emotion. Eur J Neurosci 2009;29(3):661–68,
- Parbery-Clark A, Skoe E, and Kraus N. Musical experience limits the degradative effects of background noise on the neural processing of sound. J Neurosci 2009;29(45):14100–107, 10.1523/jneurosci.3256-09.2009.
- Luo Y and Waite LJ. The impact of childhood and adult ses on physical, mental, and cognitive well-being in later life. J Gerontol B Psychol Sci Soc Sci 2005;60(2):S93–S101, 10.1093/geronb/60.2.S93.
- Law LN and Zentner M. Assessing musical abilities objectively: construction and validation of the profile of music perception skills. PLoS One 2012;7(12):e52508, 10.1371/journal.pone.0052508.
- Mankel K and Bidelman GM. Inherent auditory skills rather than formal music training shape the neural encoding of speech. Proc Natl Acad Sci U S A 2018;115(51):13129–134, 10.1073/pnas.1811793115.
- Strait DL, et al., Specialization among the specialized: Auditory brainstem function is tuned in to timbre. Cortex 2012;48(3):360–62. https://doi.org/10.1016/j.cortex.2011.03.015.
- Münte TF, et al. Specialization of the specialized: Electrophysiological investigations in professional musicians. Ann N Y Acad Sci 2003;999(1):131–39, 10.1196/annals.1284.014.
- Parbery-Clark A, et al. Musical experience and the aging auditory system: Implications for cognitive abilities and hearing speech in noise. Plos ONE 2011;6(5):e18082,
- Parbery-Clark A, et al. Musician enhancement for speech-in-noise. Ear Hear 2009;30(6):653–61.
- Strait DL, et al. Musical training during early childhood enhances the neural encoding of speech in noise. Brain Lang 2012;123(3):191–201.
- Ruggles DR, Freyman RL and Oxenham AJ. Influence of musical training on understanding voiced and whispered speech in noise. PLOS ONE 2014;9(1):e86980, 10.1371/journal.pone.0086980.
- Escobar J, Mussoi BS and Silberer AB. The effect of musical training and working memory in adverse listening situations. Ear Hear 2020;41(2):278–88. 10.1097/aud.0000000000000754.
- Slater J, et al. Music training improves speech-in-noise perception: Longitudinal evidence from a community-based music program. Behav Brain Res 2015;291:244–52. 10.1016/j.bbr.2015.05.026.
- Lo CY, et al. Music training for children with sensorineural hearing loss improves speech-in-noise perception. J Speech Lang Hear Res 2020;63(6):1990–2015. 10.1044/2020_jslhr-19-00391.