The 21st Century Has Taught Us a Great Deal About Noise-Induced Hearing Loss
In 2006, I was the co-author of a review paper on noise-induced hearing loss (NIHL) along with the late Don Henderson and Drs. Kelly C. Harris and Bo Hua Hu. The three of us as co-authors were Dr. Henderson’s graduate students, and Dr. Harris and Dr. Hu have gone on to very accomplished and impactful careers in the nearly two decades since ‘The role of oxidative stress in noise-induced hearing loss’ was published in Ear and Hearing. Reflecting back on that article, it is startling to think about how much has changed in the area of NIHL, but also how much of what we knew in the early part of the century is still relevant today.
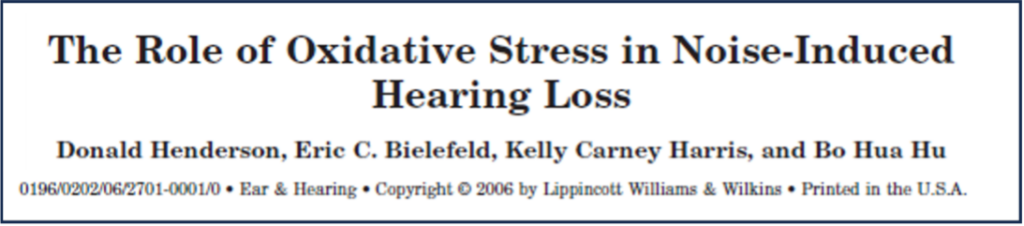
The core of the review article was an assessment of how the outer hair cells (OHCs) die as a result of high-intensity noise exposure. The focus was on two aspects: the production of reactive oxygen species (ROS) in the cochlea during and after noise exposure and the predominance of apoptosis as the mechanism through which the hair cells die. Both of those discussions offered a note of optimism that there were emerging candidate molecular pathways that could be targeted pharmaceutically to prevent the hair cells from dying and prompt them to repair and survive. To this day, the key takeaways from the article – that noise induces oxidative stress and acts as a trigger for apoptosis – still hold up as major mechanistic pathways in OHC loss underlying NIHL. The other noted pathologies of lateral wall damage (Yamaguchi et al., 2014), stereocilia dysfunction (Tsuprun et al., 2003), blood flow changes (Prazma et al., 1987), and supporting cell damage (Bohne et al., 2017) also continue to stand as important parts of the complex story about the injuries to the cochlea that can be caused by noise.
Pharmaceutical Protection from NIHL
With the clear involvement of oxidative stress in hair cell death from noise, intervening with antioxidants to mitigate oxidative stress was identified as possible way to make the ear more resistant to noise injury and resulting threshold shift. Stretching back into the late 1990’s, there have been dozens of pre-clinical research articles showing that treatment with antioxidants before a noise exposure could render the ear less susceptible to injury (Seidman et al., 1993; Quirk et al., 1994; Yamasoba et al., 1999; Kopke et al., 2000; 2002; Lynch et al., 2004; Bielefeld et al., 2005; 2007; Campbell et al., 2007; Claussen et al., 2013). Even dosing in a ‘rescue’ paradigm, in which the antioxidants are delivered after the noise exposure to relieve some of the stress on the cochlea, have proven to reduce noise-induced hair cell loss and threshold shift in pre-clinical animal models of NIHL (Campbell et al., 2011, Chen et al., 2020). Prophylactic protection paradigms have been consistently more effective, but rescue reduced the injury as well, just to a lesser degree.
Several antioxidant compounds moved forward to clinical trials (Le Prell, 2022), but the U.S. Food and Drug Administration (FDA) has yet to approve any drugs for prevention of NIHL. There have been several barriers to clinical implementation of protective drugs for NIHL. In pre-clinical animal models, investigators can plan the noise exposure and titrate the noise dose. This allows for scheduled drug treatments before, during, and after noise exposure intervals. In humans, many noise exposures occur unexpectedly (e.g. many military combat situations that arise suddenly), or they occur at regular intervals repeated for hours over months or years (e.g. occupational exposures or recreational use of personal listening devices). To protect against these exposures, one would need to take the drug compound regularly for potentially decades. There is no pre-clinical modeling or clinical trial that can fully capture the potential side effects of dosing with compounds over that long a period of time.
Clinical trials must also confront the very high variability inherent in any study of human NIHL. Due to ethical concerns, humans cannot be exposed to controlled noises known to cause NIHL. Instead, clinical trials must assess humans who are routinely being exposed to noise, often in the workplace. Those humans are often using hearing protection devices (as we would want them to). Beyond that, many NIHLs take years to manifest in measurable threshold shift. Further, inter-subject variability in susceptibility is extremely high. All of these combine, from a research standpoint, to make clinical trials of pharmaceutical protection from NIHL very challenging to design and conduct with adequate statistical power.
Finally, in addition to the duration of drug delivery, one of the great challenges in pharmaceutical protection from noise is accessing the cochlea. Local delivery of antioxidants into the cochlea before a planned noise exposure is useful in animal models for proof of concept of the protective effectiveness of a compound, but it is not currently a viable delivery method for human NIHL. Even injection of drugs into muscles or under the skin is not a reasonable option for people who would need to take them daily for years. Oral dosing is the most reasonable option. However, with oral dosing, many compounds are not orally bioavailable, meaning that they do not get absorbed into the bloodstream when ingested orally. For those that are orally bioavailable, only a small proportion of the compound enters the bloodstream. From the bloodstream, the compound must cross the blood-labyrinth barrier to enter the cochlea. This litany of obstacles has prevented compounds from making the transition from effectiveness in pre-clinical models to success in clinical trials and, ultimately, clinical implementation.
To summarize the progress on protection from NIHL, the field remains in a ‘to be continued’ phase of development. The groundbreaking findings that we summarized in the Ear and Hearing article in 2006 have opened up important paths for research and development. Important obstacles remain, but this is an important area. We know that NIHL is preventable, and pharmaceutical protection has too much potential upside for these obstacles to deter progress in the area.
Thinking Beyond Oxidative Stress
While the focus of our article was on noise-induced oxidative stress, the molecular pathways that can damage the cochlea are far broader and more complex than simply ROS formation. We touched on mechanical injury in the organ of Corti, but the impact of disruption of the precise mechanical architecture of the cochlea cannot be overstated. Metabolically, a major role for reactive nitrogen species (RNS) has been identified (Han et al., 2013), as have numerous inflammatory pathways in the organ of Corti (Frye et al., 2019; Shin et al., 2022). The role of the immune system has also been made much clearer in the nearly 20 years since the article was written. Several studies have shown that macrophage cells migrate to the sites of inflammation in the cochlea (Rai et al., 2020; Verdoodt et al., 2021; Shin et al., 2022), and they play an important role in clearing the injury site of dead or dying cell fragments. We also see the role that supporting cells play. For example, when an OHC dies, it would theoretically leave a hole in the reticular lamina that separates the endolymph from the cortilymph. However, the Deiters cells’ extend new phalangeal processes out and up to fill in the space left by the deceased OHC. This keeps the reticular lamina sealed, which is crucial for the mechanical movement of the basilar membrane to evoke horizontal deflection of the stereocilia, but also for keeping the ionic and electrical environments of the endolymph and cortilymph separate in order to facilitate rapid depolarization and then clearing of the hair cells.
Hidden Hearing Loss
Today, there is much clearer picture available of the events taking place inside the cochlea that has been exposed to high-level noise, and how those events result in the changes in thresholds that we associate with noise. When we wrote the 2006 paper, it was predicated on the notion that hair cell damage/loss leading to permanent threshold shift (PTS) was the primary concern associated with NIHL. Essentially, if you did not have PTS, you did not have NIHL. Clinically, if I diagnosed someone with temporary threshold shift (TTS) from which there was a full recovery of thresholds, my message to the patient was that they flirted with danger, but they emerged uninjured. They need to be careful in the future, but they got away with it this time. If anything, the research on sound conditioning (Ryan et al., 1994; Harrison et al., 2022) indicated that the TTS may have made the ear stronger and more resistant to future PTS. Shortly after the 2006 article was published, that paradigm was completely upended. Two seminal papers on NIHL were published in 2006 and 2009 by Sharon Kujawa and M. Charles Liberman (Kujawa and Liberman 2006; 2009). They discussed the phenomenon of cochlear synaptopathy and hidden hearing loss. In the 2006 article, they demonstrated the toxicity of early noise exposure combined with long-term aging in the mouse. That aging pattern was less impactful to the OHCs and far more impactful to the spiral ganglion neurons (SGNs), with significant evidence of loss of those neurons over time. Aging alone did not damage the SGNs this way, nor did acute noise exposure at a young or old age (Kujawa and Liberman, 2006). This finding was groundbreaking in that it pointed to the SGNs as a possible target underlying permanent noise damage. In our Ear and Hearing review, we touched on the inner hair cell-SGN synapses as a point of vulnerability for TTS, but we knew of little evidence at that time that this noise injury could cause permanent damage to the auditory system. The Kujawa and Liberman article disrupted that notion. Further, the model of neural damage from the combination of noise plus aging in the mouse mirrored what the audiology clinical community had been seeing for decades in humans. People with NIHL at a young age who then aged into the geriatric phase of the life span were showing significant speech perception impairments, particularly in challenging listening situations like in competing background noise. A version of neural presbycusis (Schuknecht and Gacek, 1993) in which there was pointed damage to the SGNs resulting from the combination of noise and aging explained a great deal of what the audiologists were seeing anecdotally in their clinics.
The groundbreaking article in 2006 was followed by another, equally impactful, one in 2009. In this article, Kujawa and Liberman saw acute damage to the ribbon synapses between the inner hair cells and SGN dendrites after a noise that causes only TTS. Even though thresholds recovered, supra-threshold ABR wave 1 amplitudes did not completely recover. These findings accomplished several important things to advance this area of research in NIHL. First, it implicated the synapses (‘cochlear synaptopathy’) as the cause of this long-term injury from noise. Instead of only contributing to TTS, synapse injury was causing long-term hearing impairment. Second, the findings implicated noise doses that cause only TTS as potentially capable of causing permanent NIHL, however, this NIHL did not manifest as threshold shift. Instead, it would manifest as difficulty hearing complex sounds, like speech, in competing listening situations (thus, name ‘hidden hearing loss’ since conventional audiometry would not be likely to capture this injury). Finally, it shortened the time interval. Synaptopathy might cause long-term death of SGNs over months in the mouse, but the loss of synapses after a single noise exposure could be contributing right away to reduced ability to understand complex sounds in challenging background noise. Again, this was (as is) very a common complaint among people with sensorineural hearing loss, and this mouse modeling was offering a very logical explanation for why it was happening.
To explain how the cochlea could sustain a noise injury that damaged complex sound perception without significant PTS, the answer lies with the spontaneous rate (SR) of the SGNs whose synapses are preferentially damaged by noise. It is low-SR neurons that sustain the most permanent synaptopathy (Furman et al., 2013), and these neurons are the ones with the highest thresholds. By contrast, high-SR neurons do not sustain nearly as much synapse injury from noise. When assessing thresholds, the presentation level of the stimulus is, by definition, very low. Therefore, threshold assessments are leveraging high-SR neurons, but largely ignoring low-SR neurons. However, when listening to speech in background noise, the noise is saturating the high-SR neurons, and the low-SR neurons must be leveraged to perceive the speech signal above that background noise. When low-SR neurons are damaged, that complex signal perception in background noise is going to be where the problems manifest.
The search continues for a differential diagnostic test for cochlear synaptopathy in the human. Despite the absence of such a test, this pathophysiology has gained widespread acceptance as a major consequence of noise and a significant contributor to the symptom of impaired speech perception in background noise that many patients with age-related and noise-induced hearing losses describe. This discovery stands as the most important evolution in the study of NIHL over the last 20 years.
Conclusion
Unsurprisingly, the last 20 years have yielded huge steps forward in our understanding of the phenomenon of NIHL. As we learn more about the cochlear pathophysiology underlying NIHL, the better equipped we are becoming in our ability to identify targets of therapeutic intervention. While the prospect of pharmaceutical treatment for NIHL was top of mind in 2006, we have entered a new era in which gene therapy approaches are being successfully deployed to treat genetic hearing losses. Future interventions to repair or replace cells damaged by noise or to restore lost synapses between hair cells and SGNs are exciting avenues to explore, and I remain hopeful that there will be clinical options for reversing noise-induced threshold shift and hidden hearing loss for patients in the future. In the meantime, the classical approaches to NIHL prevention with education, monitoring, and hearing protection devices remain effective interventions to prevent NIHL. A clever and innovative audiology community engaged and active in hearing conservation is the best defence against NIHL we have at our disposal. I expect that to continue to be true for the next 20 years as well.
References
- Bielefeld, E.C., Hynes, S., Pryznosch, D., Coleman, J.K.M., Liu, J., Henderson, D. 2005. A comparison of the protective effects of systemic administration of a pro-glutathione drug and a Src-PTK inhibitor. Noise Health, 7, 24-30.
- Bielefeld, E.C., Kopke, R. D., Jackson, R. L., Coleman, J. K., Liu, J., Henderson, D. 2007. Noise protection with N-acetyl-l-cysteine (NAC) using a variety of noise exposures, NAC doses, and routes of administration. Acta Otolaryng, 127, 914-19.
- Bohne, B.A., Kimlinger, M., Harding, G.W. 2017. Time course of organ of Corti degeneration after noise exposure. Hear Res, 344, 158-169
- Campbell K., Claussen, A., Meech, R., Verhulst, S., Fox, D., Hughes, L. 2011. D-methionine (D-met) significantly rescues noise-induced hearing loss: timing studies. Hear Res, 282, 138-44.
- Campbell. K.C., Meech, R.P., Klemens, J.J., Gerberi, M.T., Dyrstad, S.S., Larsen, D.L., Mitchell, D.L., El-Azizi, M., Verhulst, S.J., Hughes, L.F. 2007. Prevention of noise- and drug-induced hearing loss with D-methionine. Hear Res, 226, 92-103.
- Chen, G.D., Daszynski, D.M., Ding, D., Jiang, H., Woolman, T., Blessing, K., Kador, P.F., Salvi R. Novel oral multifunctional antioxidant prevents noise-induced hearing loss and hair cell loss. Hear Res, 388, 107880.
- Claussen, A.D., Fox, D.J., Yu, X.C., Meech, R.P., Verhulst, S.J., Hargrove, T.L., Campbell, K.C. 2013. D-methionine pre-loading reduces both noise-induced permanent threshold shift and outer hair cell loss in the chinchilla. Int J Audiol., 52, 801-7.
- Frye, M.D., Ryan, A.F., Kurabi, A. 2019. Inflammation associated with noise-induced hearing loss. J Acoust Soc Am, 146, 4020.
- Furman, A.C., Kujawa, S.G., Liberman, M.C. 2013. Noise-induced cochlear neuropathy is selective for fibers with low spontaneous rates. J Neurophysiol, 110, 577-86.
- Han, W.J., Shi, X.R., Nuttall, A. 2013. Noise-induced nitrotyrosine increase and outer hair cell death in guinea pig cochlea. Chin Med J, 126, 2923-7.
- Harrison, R.T., DeBacker, J.R., Trevino, M., Bielefeld, E.C., Lobarinas, E. (2022). Cochlear Preconditioning as a Modulator of Susceptibility to Hearing Loss. Antioxid Redox Signal, 36, 1215-28.
- Henderson, D., Bielefeld, E.C., Harris, K.C., Hu, B.H. 2006. The Role of Oxidative Stress in Noise-induced Hearing Loss. Ear Hear, 27, 1-19.
- Kopke, R. D., Coleman, J. K., Liu, J., Campbell, K. C., Riffenburgh, R. H. 2002. Candidate's thesis: enhancing intrinsic cochlear stress defenses to reduce noise-induced hearing loss. Laryngoscope, 112, 1515-32.
- Kopke, R.D., Weisskopf, P.A., Boone, J.L., Jackson, R.L., Wester, D.C., Hoffer, M.E., Lambert, D.C., Charon, C.C., Ding, D.L., McBride, D. 2000. Reduction of noise-induced hearing loss using L-NAC and salicylate in the chinchilla. Hear Res, 149, 138-46.
- Kujawa, S.G., Liberman, M.C. 2006. Acceleration of age-related hearing loss by early noise exposure: evidence of a misspent youth. J Neurosci, 26, 2115-23.
- Kujawa, S.G., Liberman, M.C. 2009. Adding insult to injury: cochlear nerve degeneration after "temporary" noise-induced hearing loss. J Neurosci, 29, 14077-85.
- Le Prell, C.G. 2022. Prevention of Noise-Induced Hearing Loss Using Investigational Medicines for the Inner Ear: Previous Trial Outcomes Should Inform Future Trial Design. Antioxid Redox Signal, 36, 1171-202
- Lynch, E.D., Gu, R., Pierce, C., Kil, J. 2004. Ebselen-mediated protection from single and repeated noise exposure in rat. Laryngoscope, 114, 333-7.
- Prazma, J., Vance, S.G., Bolster, D. E., Pillsbury, H. C., Postma, D. S. 1987. Cochlear blood flow. The effect of noise at 60 minutes' exposure. Arch Otolaryngol Head Neck Surg, 113, 36-9.
- Quirk, W.S., Shivapuja, B.G., Schwimmer, C.L., Seidman, M.D. 1994. Lipid peroxidation inhibitor attenuates noise-induced temporary threshold shifts. Hear Res, 74, 217-20.
- Rai, V., Wood, M.B., Feng, H., Schabla, N.M., Tu, S., Zuo, J. 2020. The immune response after noise damage in the cochlea is characterized by a heterogeneous mix of adaptive and innate immune cells. Sci Rep, 10, 15167.
- Ryan, A.F., Bennett, T.M., Woolf, N.K., Axelsson, A. 1994. Protection from noise-induced hearing loss by prior exposure to a nontraumatic stimulus: role of the middle ear muscles. Hear Res, 72, 23-8.
- Seidman, M.D., Shivapuja, B.G., Quirk, W.S. 1993. The protective effects of allopurinol and superoxide dismutase on noise-induced cochlear damage. Otolaryngol Head Neck Surg, 109, 1052-6.
- Shin, S.H., Yoo, J.E., Jung, J., Choi, J.Y., Bae, S.H. 2022. Inflammatory Monocytes Infiltrate the Spiral Ligament and Migrate to the Basilar Membrane After Noise Exposure. Clin Exp Otorhinolaryngol, 15, 153-9.
- Schuknecht, H.F., Gacek, M.R. 1993.Cochlear pathology in presbycusis. Ann Otol Rhinol Laryngol, 102, 1-16.
- Tsuprun V, Schachern PA, Cureoglu S, Paparella M. 2003. Structure of the stereocilia side links and morphology of auditory hair bundle in relation to noise exposure in the chinchilla. J Neurocytol, 32, 1117-28.
- Verdoodt, D., Peeleman, N., Szewczyk, K., Van Camp, G., Ponsaerts, P., Van Rompaey, V. 2021. Cochlin Deficiency Protects Aged Mice from Noise-Induced Hearing Loss. Int J Mol Sci, 22, 11549.
- Yamaguchi, T., Nagashima, R., Yoneyama, M., Shiba, T., Ogita., K. 2014. Disruption of ion-trafficking system in the cochlear spiral ligament prior to permanent hearing loss induced by exposure to intense noise: possible involvement of 4-hydroxy-2-nonenal as a mediator of oxidative stress. PLOS One, 9, e102133.
- Yamasoba, T., Schacht, J., Shoji, F., Miller, J.M. 1999. Attenuation of cochlear damage from noise trauma by an iron chelator, a free radical scavenger and glial cell line-derived neurotrophic factor in vivo. Brain Res, 815, 317-25.