The “No Longer Hidden” Hearing Loss and Audiology: Bridging the Research-Clinic Gap
You sit your patient down to obtain case history information prior to starting a comprehensive evaluation. They report significant difficulty hearing in background noise, which has a negative influence on their work and social life. During the audiometric evaluation, you obtain completely normal air and bone conduction thresholds and excellent word discrimination ability in quiet. The patient is counselled on the results and how to utilize effective communication strategies and is sent on their way while the audiologist is left puzzled. Why was the patient genuinely experiencing such difficulty in complex listening environments with normal audiometric thresholds? Research has now uncloaked the mechanism behind this “hidden hearing loss” which has remained elusive for decades.
In the Lab
Previously, audiologists and researches believed that outer her cells (OHCs) were the most susceptible mechanism to noise- and age-related hearing loss.1 However, recent research utilizing animal models at Harvard Medical School have discovered that the synapses communicating between inner hair cells (IHC) and afferent auditory nerve fibers are even more susceptible to damage than outer hair cells.2–4 The most interesting factor related to this new discovery is that the damage does not impair audiometric thresholds, rendering the disorder “hidden” from more traditional audiometric evaluations – primarily a neural issue rather than a sensory one. This however does not mean that the disorder lacks significant impact on an individual’s life. Many patients suffering from this “hidden” hearing loss can expect to experience increased difficulty discriminating sounds in the presence of background noise, making many work and social environments frustrating and unenjoyable.
To understand the science behind this disorder let us first briefly review the synaptic structure between IHCs and afferent auditory nerve fibers. IHCs contain bulks of protein known as pre-synaptic ribbons located at their site of communication with auditory nerve fibers. These ribbon like structures are responsible for releasing glutamate into the synaptic cleft in order to stimulate post-synaptic nerve fibres.5 Post-synaptic fibers have glutamate receptors which receive the released glutamate which stimulate the nerve to generate an action potential for further downstream decoding in the central auditory system.6 Researchers are able to visually inspect this mechanism by immunostaining protein markers on pre-synaptic ribbons (Ctbp2) and post-synaptic glutamate receptors (GluA2).2–4,7–12 This method provides a fluorescent co-localized image of the pre- and post- sections of the synaptic structure when visualized under a confocal microscope.
By using this immunostaining method, researchers have discovered that pre-synaptic ribbons disappear following noise exposures that do not generate permanent threshold shift and in typical aging animals. Furthermore, spiral ganglion neurons progressive degenerate following loss of this synaptic connection.10 This damage to the synapse has been termed “Synaptopathy” and is likely responsible for many patients perceived difficulty hearing in noise even when they have normal audiometric thresholds.
Using the immunostaining method to detect and further understand the mechanism behind synaptopathy is a great tool for basic researchers. However, its flaw is that it can only be performed in post-mortem subjects. Fortunately, physiologic measures of the neural output of the cochlea can be used to evaluate the presence of synaptopathy instead. Auditory brainstem response (ABR) and/or electrocochleography (EcoG) can both show neural-representations of auditory nerve activity and detect the presence of this acquired impairment. If synaptopathy is present you would expect to see a reduction in amplitude (e.g., wave amplitude in neurological response) in the compound action potential (CAP) or Wave I of the ABR when stimulated at supra-threshold levels while functional measurements of the outer hair cells (e.g., DPOAEs) remain normal. This makes physiologic observations of synaptopathy very similar, to those that are typically observed in auditory neuropathy.
Probably the most interesting phenomena associated with synaptopathy is the lack of impact on auditory thresholds. Surprisingly, even when animals are subjected to mild to moderate inner hair cell damage (30–60% loss) they continue to display normal threshold functions.13 This observation suggests that only minimal intact synapses are required to obtain normal audiometric thresholds. Despite obtaining normal thresholds, animals demonstrate impaired ability to discriminate between tones in the presence of background noise.14
How can damage to the pre-synaptic ribbons result in impaired ability to distinguish between sounds in background noise but leave thresholds unaffected? This likely results from specific synaptic damage to auditory nerve fibers which have a low spontaneous firing rate (low-SR). These Low-SR nerve fibers are primarily important to coding sound at moderate-loud intensities, while their high spontaneous firing rate (high-SR) counterparts, responsible for coding sound at lower intensity levels, remain unaffected.8 Therefore, threshold level nerve fibers would maintain healthy synaptic connections, but auditory nerve fibers that are important for hearing in louder environments are damaged first. Being that thresholds have been the primary measurement tool for diagnosing hearing loss in clinical evaluations for decades, we can see how this has gone unnoticed.
Like all other audiological disorders, the impact of synaptopathy does not simply stop at the level of the periphery. Additional animal studies have indicated that massive loss of inner hair cells, and therefore synapses, can lead to enhanced neural gain at the levels of the auditory midbrain and auditory cortex.15 Central gain is a well-documented phenomena that occurs essentially whenever there is a reduced neural output from the peripheral system.16 This likely serves as a neural compensatory phenomena for those individuals with a mild-moderate hearing loss, however, if the gain becomes dysregulated it can result in additional auditory perceptual disorders such as tinnitus and hyperacusis.16 Therefore, synaptopathy may also serve as one of the elusive mechanisms responsible for the generation of tinnitus and/or hyperacusis.
In the Clinic
After years of investigation, this “more susceptible mechanism” research has recently turned to a clinically based approach to determine if synaptopathy also occurs in human populations. Post-mortem analysis of adult temporal bones with a known history of age-related hearing loss has confirmed that synaptopathy in fact does occur within the human population.17
Since “hidden hearing loss” primarily influences an individual’s ability to clearly understand speech in noise, incorporating some of the already developed speech-in-noise psychometric tests seem like a plausible method of evaluation. These speech-in-noise tests have been available for many years, but not broadly utilized in clinical audiology due to its lack of prognostic utility, and the clinical time required lacks feasible reimbursement. However, the field of audiology may have a new use of this measurement. Clinical research shows a high correlation between speech-in-noise scores and individuals who are classified as high-risk for “hidden” hearing loss.18 Like other audiometric tests, a crosscheck principle needs to be in place to increase the clinician’s confidence in results. Using measurements obtained from EcoG can provide this additional confidence. Results from the same clinical study indicate that individuals who are high-risk for hidden hearing loss show reduce CAP amplitudes and enhanced summating potentials (SP) amplitudes.18 One method of interpreting these electrophysiological measures is to use the SP/AP ratio. This is currently used for diagnostic utility of Meniere’s disease. However, it may also be useful in the diagnosis of hidden hearing loss since high-risk individuals show a much greater SP/AP ratio (demonstrating both a higher than normal SP and a lower than normal AP) than low-risk individuals (Table 1).
Table 1. SP/AP Ratio
In addition to the synaptopathy’s impact on hearing, it was previously noted that the damaged mechanism might also be responsible for the long elusive perception of tinnitus and/or hyperacusis. Both tinnitus and hyperacusis have been previously linked to an aberrant enhancement in central gain.16 which is also observed when damage is done specifically to the cochlear synapses.15 Clinical research in humans has further determined the likelihood that (1) tinnitus perceptions stem from enhanced central gain and that (2) damage to cochlear synapses may partially be responsible for the presence of tinnitus without measurable threshold change.19 Scheatte et al., 201119 measured ABRs from individuals reporting bothersome tinnitus who also had normal audiometric thresholds. They found that ABR wave I amplitudes were much smaller than controls (an indication of synaptopathy), and furthermore, that Wave V was significantly larger than the same controls (Figure 1). This paradoxical change in amplitudes between the periphery and auditory midbrain suggests the presence of enhanced central gain. Therefore, observing the ABR Wave I/V amplitude ratio (lower amplitude Wave I and higher amplitude Wave V resulting in a lower than normal number), may also be a good clinical indicator of synaptopathy induced tinnitus and/or hyperacusis (Table 2).
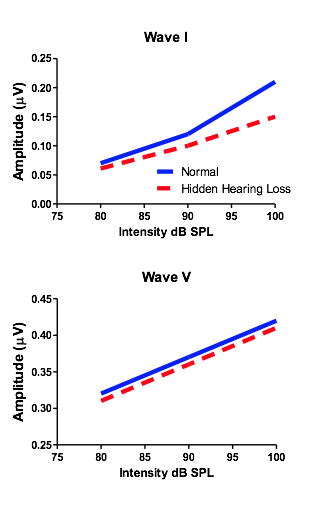
Figure 1. ABR Wave I/V amplitude ratio.
Table 2. Normal Hearing Function versus Hidden Hearing Loss
Finally, one last question remains before the discovery to treatment cycle can be completed. We now know that synaptopathy exists in the human cochlea, and that its presence likely results in significant difficulty hearing in background noise. By simply exercising already developed psychometric and electrophysiology evaluations we can determine if the disorder is presence in individual patients. However, now we responsible for discovering a method of improving the condition at hand. In 2015, The National Institutes of Health held a workshop, which essentially developed a road map to the development of treatments of synaptopathy. Currently research is continuing to explore the fine details of the mechanisms involved with the development of this disorder, therefore, there remains no cure. It remains to be explored weather or not some of the advanced processing features in modern hearing aid technology may help these individuals enhanced their ability to understand in background noise.
Conclusions
In conclusion, auditory research has now discovered and classified a new type of hearing loss which was “hidden” for decades. This new type of acquired hearing loss requires a drastic need for alterations in the test measures that are included in “comprehensive evaluations.” Luckily, the disorder can be detected with already developed and readily available clinical measures. We can now say that the “hidden hearing loss” is no longer hidden. Research has now confirmed the presence of synaptopathy in aging and noise exposed humans, and developed diagnostic tests that can detect the problem pre-mortem. It is time to remove the cloak of this hearing deficit in clinical practice and start incorporating these evaluation measures into daily patient interactions.
References
- Chen GD and Fechter LD. The relationship between noise-induced hearing loss and hair cell loss in rats. Hear Res 2003;177(1-2):81–90.
- Kujawa SG and Liberman MC. Adding insult to injury: cochlear nerve degeneration after "temporary" noise-induced hearing loss. J Neurosci 2009;29(45):14077–85.
- Kujawa SG and Liberman MC. Synaptopathy in the noise-exposed and aging cochlea: Primary neural degeneration in acquired sensorineural hearing loss. Hear Res 2015;330(Pt B):191–9.
- Kujawa SG and Liberman MC. Acceleration of age-related hearing loss by early noise exposure: evidence of a misspent youth. J Neurosci 2006;26(7):2115–23.
- Fuchs PA, Glowatzki E, and Moser T. The afferent synapse of cochlear hair cells. Curr Opin Neurobiol 2003;13(4):452–8.
- Parks TN. The AMPA receptors of auditory neurons. Hear Res 2000;147(1-2):77–91.
- Chen Z, Kujawa SG, and Sewell WF. Auditory sensitivity regulation via rapid changes in expression of surface AMPA receptors. Nat Neurosci 2007;10(10):1238–40.
- Furman AC, Kujawa SG, and Liberman MC. Noise-induced cochlear neuropathy is selective for fibers with low spontaneous rates. J Neurophysiol 2013;110(3):577–86.
- Kujawa SG and Liberman MC Long-term sound conditioning enhances cochlear sensitivity. J Neurophysiol 1999;82(2):863–73.
- Liberman LD, Suzuki J, and Liberman MC. Dynamics of cochlear synaptopathy after acoustic overexposure. J Assoc Res Otolaryngol 2015;16(2):205–19.
- Lin HW, et al., Primary neural degeneration in the Guinea pig cochlea after reversible noise-induced threshold shift. J Assoc Res Otolaryngol 2011;12(5):605–16.
- Sergeyenko Y, et al. Age-related cochlear synaptopathy: an early-onset contributor to auditory functional decline. J Neurosci 2013;33(34):13686–94.
- Lobarinas E, Salvi R, and Ding D. Insensitivity of the audiogram to carboplatin induced inner hair cell loss in chinchillas. Hear Res 2013;302:113–20.
- Lobarinas E, Salvi R and Ding D Selective inner hair cell dysfunction in chinchillas impairs hearing-in-noise in the absence of outer hair cell loss. J Assoc Res Otolaryngol 2015.
- Chambers AR, et al. Central gain restores auditory processing following near-complete cochlear denervation. Neuron 2016;89(4):867–79.
- Auerbach BD, Rodrigues PV and Salvi RJ. Central gain control in tinnitus and hyperacusis. Front Neurol 2014;5:206.
- Viana LM, et al. Cochlear neuropathy in human presbycusis: Confocal analysis of hidden hearing loss in post-mortem tissue. Hear Res 2015;327:78–88.
- Shaheen LA, Valero MD, and Liberman MC. Towards a diagnosis of cochlear neuropathy with envelope following responses. J Assoc Res Otolaryngol 2015;16(6):727–45.
- Schaette R and McAlpine D. Tinnitus with a normal audiogram: physiological evidence for hidden hearing loss and computational model. J Neurosci 2011;31(38):13452–7.