A New Perspective on Chronic Conductive Hearing Loss
Age-Related Hearing Loss (AHRL) is a simplistic way to define presbycusis, the most common type of sensorineural hearing loss in adult populations. There is abundant evidence showing that presbycusis is more than an innate genetic process whereby one continuously loses hearing with age. In humans, and in the absence of a distinct audiometric pattern (e.g., 4-kHz notch), it is nearly impossible to identify with certainty the respective contribution of ARHL and noise-induced hearing loss (NIHL) to the audiometric phenotype. Every day, particularly in urban settings, we are exposed to noise (traffic, construction, cafeteria, etc.…). While these daily exposures to noise are deemed to be “atraumatic” in the sense that they are not followed by a measurable change in hearing thresholds, it is legitimate to question whether these daily noise exposures affect our hearing health throughout a lifetime.
For many years, investigators have sought to evidence an accumulative effect of our daily exposures to noise. As early as 1962, Dr. Rosen investigated the Mabaan of South Sudan, a Nilotic tribe within sub-Saharan Africa.1 This unique aging study described how hearing thresholds from the Mabaan population changed over time compared to Wisconsinites who attended the State Fair of 1954. While the younger participants from each group had normal hearing thresholds (as measured from 500 Hz to 6,000 Hz), the older population from Wisconsin exhibited a severe hearing loss by age 70-79. To my amazement, the age-matched Mabaan group (70-79) had mean hearing thresholds no worse than 25 dB HL. By the late 19th century, factories producing agricultural gears, industrial equipment, steel, ships and boats, and other durable goods were standard in Wisconsin urban life. Manufacturing continued to dominate Wisconsin’s economy many decades later, where the manufacture of heavy machinery, tools, and engines rivaled the rural quietude encountered in the sub-Saharan desert.
Granted, the Mabaan people may have a different genetic makeup that could account for a difference in sensitivity to noise overexposure. This caveat was partly addressed by the no less intriguing findings of Dr. Goycoolea and colleagues2, who studied the effect of industrialization on hearing health. This study recruited native participants of Easter Island, one of the most isolated inhabited islands in the world. While some participants spent their entire lives on this volcanic island, some spent many years abroad in more industrialized cities in Chile. The former group presented hearing thresholds within near-normal limits, whereas thresholds from the latter group showed a moderately-severe sensorineural hearing loss. Both groups were age-matched (mean = 59 y.o.). Altogether, these studies support the idea that our environmental noise level significantly impacts hearing thresholds.
To avoid the variability inherent in human studies and dissect the contribution of daily noise doses to presbycusis, we designed a study with mice presenting with a chronic conductive hearing loss that results from the unilateral ablation of the eardrum.3 Such tympanic membrane resection creates a 30-40 dB conductive hearing loss. The idea was to develop a long-term deficit of auditory afferent inputs to one ear in hopes to see if, after waiting a long time (64 weeks or ~half of the lifespan of mice raised in a lab), we could evidence a protective effect of the conductive impairment to the inner ear.
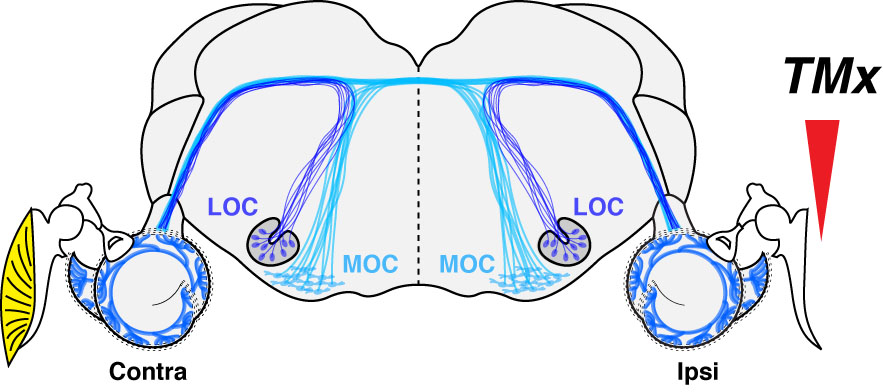
And indeed, we did see significant histological differences between ears; surprisingly, in the opposite direction of what we expected! In short, inter-aural comparisons and comparison with control mice with intact eardrums showed no effect of the conductive component on hair cell count (inner and outer); however, as much as a third of the synaptic connections between the inner hair cells and the auditory nerve were no longer visible on the side of the conductive hearing loss. Such unexpected histopathology was not related to possible ossicular chain damage resulting from the eardrum resection and indirectly impacting the inner ear as the same phenotype was observed in a few control mice with untreated otitis media.
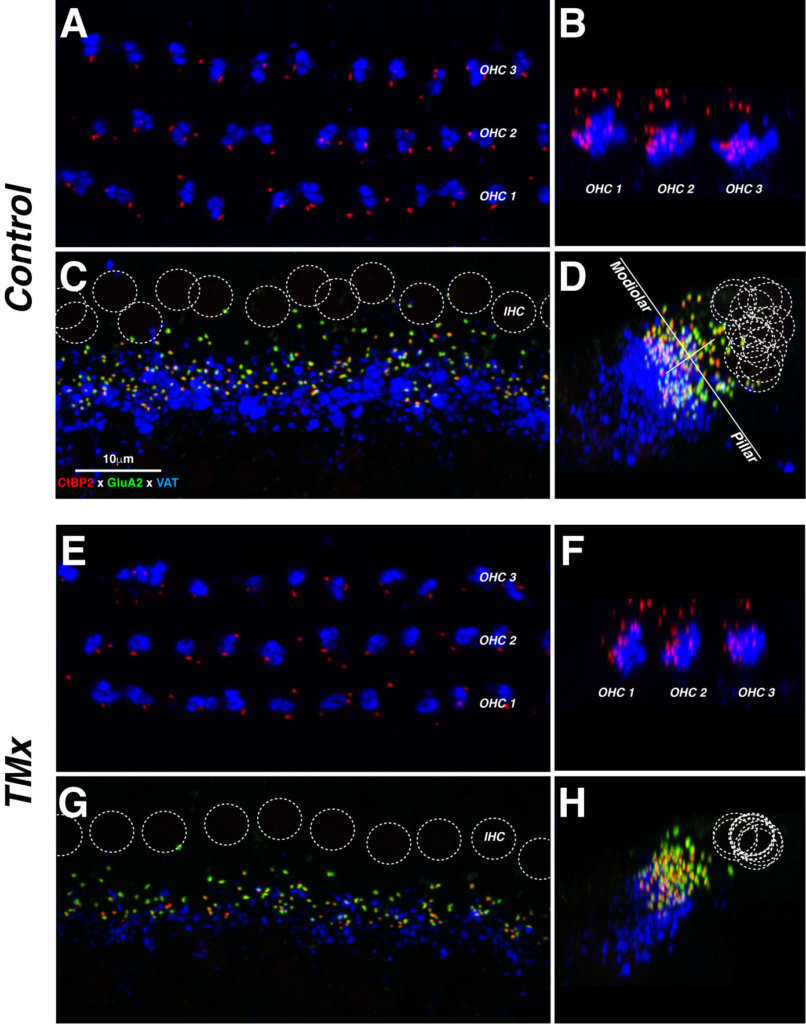
Each row of images (e.g., A, B) contains a pair of maximal projections of a confocal z-stack through 10–12 adjacent hair cells, viewed either in the acquisition plane (left) or the digitally rotated plane (right). Each afferent synapse in the inner hair cell (IHC) area (C, G,) appears as a closely apposed pair of red and green puncta. OC terminals in both IHC and outer hair cell (OHC) areas appear in the blue channel. Positions of inner hair cell nuclei are shown as dotted white circles (C, G). The white lines in D show the modiolar-pillar and habenular-cuticular axes used in the spatial analysis of IHC synapses. Scale in C applies to all micrographs from the 22 kHz region. Source: https://doi.org/10.1371/journal.pone.0142341.g005
While this twist of fate left us with unanswered questions regarding presbycusis, we did show that a chronic conductive hearing loss could lead to cochlear nerve degeneration.
This considerable loss of afferent inputs was associated with a massive loss (as much as 60%) of lateral olivocochlear (LOC) efferent terminals on the side of the chronic conductive hearing loss. Indeed, in the absence of a hair cell phenotype, we directed our attention to the efferent fibers originating from the brainstem and projecting to the cochlea. A loss of LOC efferents associated with cochlear nerve degeneration was not entirely surprising because 1) these fibers project to the dendrites of the auditory afferent fibers, and 2) we provided evidence that OC efferent fibers protect the inner ear from aging. Indeed, in mice where we accessed the brainstem near the floor of the IVth ventricle to section all efferent fibers projecting to one ear, we observed an age-related loss of auditory nerve fibers that was twice as fast as controls.4
As a result of these studies, we suggested that efferent fibers have dramatic use-dependent plasticity in adult mice as a loss of auditory input leads to a degeneration of LOC efferent fibers; in turn, a loss of LOC efferent fibers leads to cochlear nerve degeneration.
We were very fortunate to have the records of 240 patients who presented with an acute or chronic unilateral conductive hearing loss.5 We observed that if the conductive component was chronic and associated with at least a moderate to moderately-severe hearing loss, speech recognition scores were significantly lower on the side of the conductive hearing loss even if bone conduction thresholds remain symmetrical within normal limits bilaterally. Cochlear nerve degeneration is thought to cause hearing impairments, especially in noisy environments,6 because the most vulnerable cochlear neurons to both noise and aging are those with high thresholds and low-spontaneous rates.7 To date, several studies have shown poorer temporal processing and signal-in-noise detection task performances in animals with cochlear nerve degeneration8–11 but also in humans with reduced ABR Wave 1 amplitude, larger SP amplitude, or larger SP/AP ratio12–16 or reduced EFR magnitudes.17
As audiologists dealing with pediatric populations, we know full well that chronic conductive hearing loss is not infrequent. 80% of children before age 3 experience an ear infection. These bouts can persist for many months, and deficits in spatial hearing and receptive language skills can persist for years after the middle-ear pathology has resolved.18 Given that cochlear nerve degeneration appears to be irreversible, peripheral deficits should be considered when patients report lingering deficits in auditory processing after persistent middle-ear issues are resolved. Our studies also support the idea that amplification should be considered in managing unilateral conductive hearing loss if hearing cannot be medically improved. Our data suggest that speech recognition, particularly in adverse environments, may worsen on the side of the pathology. This hypothesis is supported by a study showing that speech-recognition in unaided ears was poorer than in aided ears in patients with bilateral and symmetric conductive hearing loss.19
References
- Rosen, S., Bergman, M., Plester, D., El-Mofty, A., and Hamad Satti, M. (1962). “Presbycusis study of a relatively noise-free population in the Sudan,” Transactions of the American Otological Society 50, 135-152.
- Goycoolea, M. V., Goycoolea, H. G., Farfan, C. R., Rodriguez, L. G., Martinez, G. C., and Vidal, R. (1986). “Effect of life in industrialized societies on hearing in natives of Easter Island,” Laryngoscope 96, 1391-1396.
- Liberman, M. C., Liberman, L. D., and Maison, S. F. (2015). “Chronic Conductive Hearing Loss Leads to Cochlear Degeneration,” PloS one 10, e0142341.
- Liberman, M. C., Liberman, L. D., and Maison, S. F. (2014). “Efferent feedback slows cochlear aging,” J Neurosci 34, 4599-4607.
- Okada, M., Welling, D. B., Liberman, M. C., and Maison, S. F. (2020). “Chronic Conductive Hearing Loss Is Associated With Speech Intelligibility Deficits in Patients With Normal Bone Conduction Thresholds,” Ear and hearing 41, 500-507.
- Liberman, M. C. (2017). “Noise-induced and age-related hearing loss: new perspectives and potential therapies,” F1000Res 6, 927.
- Furman, A. C., Kujawa, S. G., and Liberman, M. C. (2013). “Noise-induced cochlear neuropathy is selective for fibers with low spontaneous rates,” Journal of neurophysiology 110, 577-586.
- Chambers, A. R., Resnik, J., Yuan, Y., Whitton, J. P., Edge, A. S., Liberman, M. C., and Polley, D. B. (2016). “Central Gain Restores Auditory Processing following Near-Complete Cochlear Denervation,” Neuron 89, 867-879.
- Lobarinas, E., Salvi, R., and Ding, D. (2020). “Gap Detection Deficits in Chinchillas with Selective Carboplatin-Induced Inner Hair Cell Loss,” J Assoc Res Otolaryngol 21, 475-483.
- Monaghan, J. J. M., Garcia-Lazaro, J. A., McAlpine, D., and Schaette, R. (2020). “Hidden Hearing Loss Impacts the Neural Representation of Speech in Background Noise,” Current biology : CB.
- Resnik, J., and Polley, D. B. (2021). “Cochlear neural degeneration disrupts hearing in background noise by increasing auditory cortex internal noise,” Neuron 109, 984-996 e984.
- Bramhall, N., Ong, B., Ko, J., and Parker, M. (2015). “Speech Perception Ability in Noise is Correlated with Auditory Brainstem Response Wave I Amplitude,” Journal of the American Academy of Audiology 26, 509-517.
- Liberman, M. C., Epstein, M. J., Cleveland, S. S., Wang, H., and Maison, S. F. (2016). “Toward a Differential Diagnosis of Hidden Hearing Loss in Humans,” PloS one 11, e0162726.
- Ridley, C. L., Kopun, J. G., Neely, S. T., Gorga, M. P., and Rasetshwane, D. M. (2018). “Using Thresholds in Noise to Identify Hidden Hearing Loss in Humans,” Ear and hearing 39, 829-844.
- Buran, B. N., McMillan, G., Keshishzadeh, S., Verhulst, S., and Bramhall, N. (2020). “Predicting synapse counts in living humans by combining computational models with auditory physiology.,” OSF Preprints.
- Grant, K. J., Mepani, A. M., Wu, P., Hancock, K. E., de Gruttola, V., Liberman, M. C., and Maison, S. F. (2020). “Electrophysiological markers of cochlear function correlate with hearing-in-noise performance among audiometrically normal subjects,” J Neurophysiol 124, 418-431.
- Mepani, A. M., Verhulst, S., Hancock, K. E., Garrett, M., Vasilkov, V., Bennett, K., de Gruttola, V., Liberman, m. c., and Maison, S. F. (2021). “Envelope following responses predict speech-in-noise performance in normal hearing listeners.,” J Neurophysiol 125, in press.
- Deggouij, N., Castelein, S., Grégoire, A., Laroche, H., De Graeuwe, C., and De Toeuf, C. (2012). “Functional consequences of chronic ENT inflammation on the development of hearing and communcative abilities.,” B-ENT 19, 105-115.
- Dieroff, H. G. (1993). “Late-onset auditory inactivity (deprivation) in persons with bilateral essentially symmetric and conductive hearing impairment,” Journal of the American Academy of Audiology 4, 347-350.
Figures reprinted with permission:
Citation: Liberman MC, Liberman LD, Maison SF (2015) Chronic Conductive Hearing Loss Leads to Cochlear Degeneration. PLoS ONE 10(11): e0142341. https://doi.org/10.1371/journal.pone.0142341
Editor: Olivia Bermingham-McDonogh, University of Washington, Institute for Stem Cells and Regenerative Medicine, UNITED STATES
Received: September 1, 2015; Accepted: October 20, 2015; Published: November 18, 2015
Copyright: © 2015 Liberman et al. This is an open access article distributed under the terms of the Creative Commons Attribution License, which permits unrestricted use, distribution, and reproduction in any medium, provided the original author and source are credited.