Tinnitus with a Normal Audiogram
Is Hearing Damage Missed by Conventional Testing?
Most cases of chronic, subjective tinnitus are associated with hearing damage due to noise exposure, age-related hearing loss, ototoxic drugs, or other trauma. With hearing damage, auditory neurons in the brain no longer receive the input to which they are accustomed from the ear, and through mechanisms of neural plasticity, become hyperexcitable.1 Their rate of spontaneous activity increases, they respond more strongly to sound input, and groups of neurons start firing more synchronously together and inhibit each other less.2 These hyperactive patterns have been observed to extend from the brainstem to the auditory cortex, and altered activity has also sometimes been found in networks involved in consciousness, cognition, and emotion.3 Some or all of these neural changes can manifest as the experience of chronic ringing, as well as a person’s reaction to it. This view summarizes a leading, but potentially incomplete, understanding of how subjective tinnitus may occur.
Consider a circumstance that many clinicians have likely experienced: A patient presents with bothersome tinnitus, but their audiogram is within normal limits of 8 kHz in both ears. If the biggest risk factor for tinnitus is hearing loss, how might we explain this case?
Before addressing possible answers, it is necessary to mention that there are likely cases of tinnitus that are entirely idiopathic and not associated with the status or integrity of the ear. However, for the 8% to 15% of individuals with tinnitus who do not have threshold shifts in the audiogram,4,5 there is likely a hearing-related cause for their tinnitus.
This article outlines evidence in support of this theory, describing three ways by which sensorineural hearing damage can “hide” from conventional pure-tone audiometry. (In this article, “conventional pure-tone audiometry” refers to behavioural testing for thresholds of audibility at octave steps to a maximum of 8 kHz.)
Low Precision in Conventional Audiometry Misses Threshold Shifts
We have long known that conventional pure-tone audiometry does not paint a complete picture of a patient’s hearing and often does not relate to real-world listening problems.6 The audiogram is not a poor measure, but it provides a coarse view of frequencies at which there can be hearing damage to the extent that audibility thresholds are elevated. One way that conventional audiometry can miss evidence of hearing loss involves low precision (Figure 1).
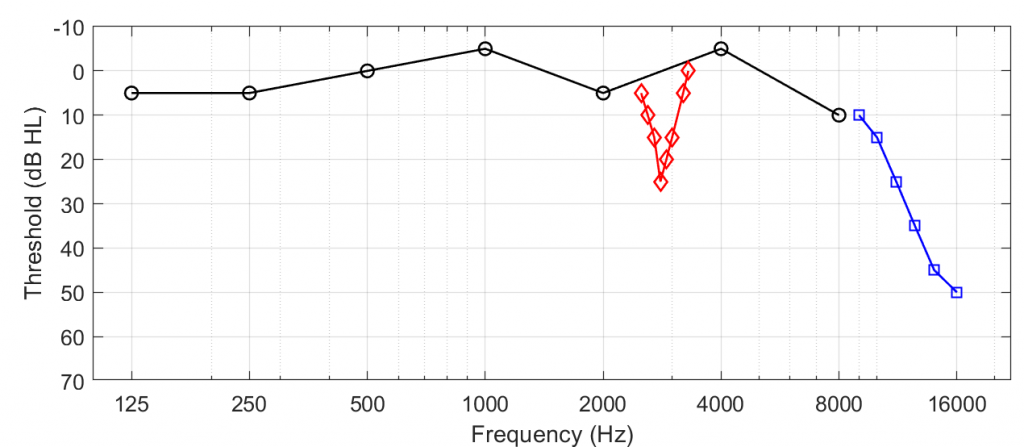
For instance, when testing at octave frequencies, it is possible to miss notches at inter-octave steps that reflect frequency-restricted hearing damage. In a clever demonstration, Xiong et al.7 recruited 106 patients with normal conventional audiograms and used a psychophysical procedure to estimate the pitch at which each person experienced their tinnitus. They then measured thresholds at 1/24-octave steps above and below the tinnitus pitch. Remarkably, 52 of their 106 participants had notched threshold shifts in the tested ear that were proximal to each individual’s tinnitus pitch. Thus, by increasing the precision to threshold testing, the experimenters identified evidence of hearing loss concealed between the conventional inter-octave test frequencies.
A second way that conventional audiometry can miss threshold shifts is that it doesn’t test frequencies above 8 kHz, sometimes referred to as the extended high-frequency region. Several studies (e.g., one by Vielsmeier et al.8) have found that individuals with tinnitus and normal conventional audiograms have shifts greater than 20 dB HL above 8 kHz. Thus, when extended high frequencies are not tested, threshold shifts can be missed. Note that threshold shifts between 8 and 16 kHz are not diagnostic of tinnitus-related hearing loss. Many individuals with tinnitus have normal thresholds within these high-frequency regions.5,9
Presence of Cochlear “Dead Regions” Despite Normal Audiograms
Animal experiments in the past decade have revealed that inner hair cells of the cochlea, responsible for converting mechanical sound energy into neural signals that are passed to the brain via the auditory nerve, can sustain a wide degree of loss (~80%) before audiometric thresholds increase.10 It might be surprising that such a degree of hearing damage is possible without detection by an audiogram, but this finding also highlights the resilience of the auditory system. Could selective inner hair cell loss describe a form of hearing damage related to tinnitus?
This question was addressed by Weisz et al.,11 who probed for evidence of “dead regions,” or places along the cochlea with non-functioning inner hair cells, in people experiencing tinnitus. To detect dead regions, the authors used a psychophysical pitch task as well as the Threshold Equalizing Noise (TEN) test (see also “Testing for Cochlear Dead Regions: Audiometer Implementation of the TEN(HL) Test,” in Canadian Audiologist, Volume 7, Issue 4, 2020). Weisz and colleagues found evidence of dead regions in eight of 11 individuals with tinnitus who otherwise had normal audiograms. The authors concluded that dead regions may be a type of damage that precedes the neuroplastic changes associated with tinnitus. However, attempts to replicate this report have shown different results. One study found no evidence of dead regions via the TEN test in 33 people with tinnitus,12 and this agreed with another report that found a better association between otoacoustic emissions and tinnitus than with dead regions.13 (Note that outer hair cell loss as evidenced by otoacoustic emissions has not been reliably associated with tinnitus.14) Thus, while we should be aware that dead regions could be a form of cochlear damage that arises in some people with tinnitus, these dead regions are not a predictor of tinnitus.
Cochlear Synaptopathy: Disconnecting the Hair Cell from the Auditory Nerve
Research in animal models by Sharon Kujawa and Charles Liberman in the mid- to late 2000s challenged the view that noise-induced “temporary” audiometric threshold shifts are innocuous and leave no long-lasting effects on hearing.15 These authors found that moderate noise exposure that did not permanently elevate thresholds produced an irreversible loss of cochlear ribbon synapses, which are a part of small junctions that connect inner hair cells to auditory nerve fibres (Figure 216). Referred to as cochlear synaptopathy, this form of synaptic losspreceded the eventual death of spiral ganglion cells of the auditory nerve many months after the initial noise exposure.
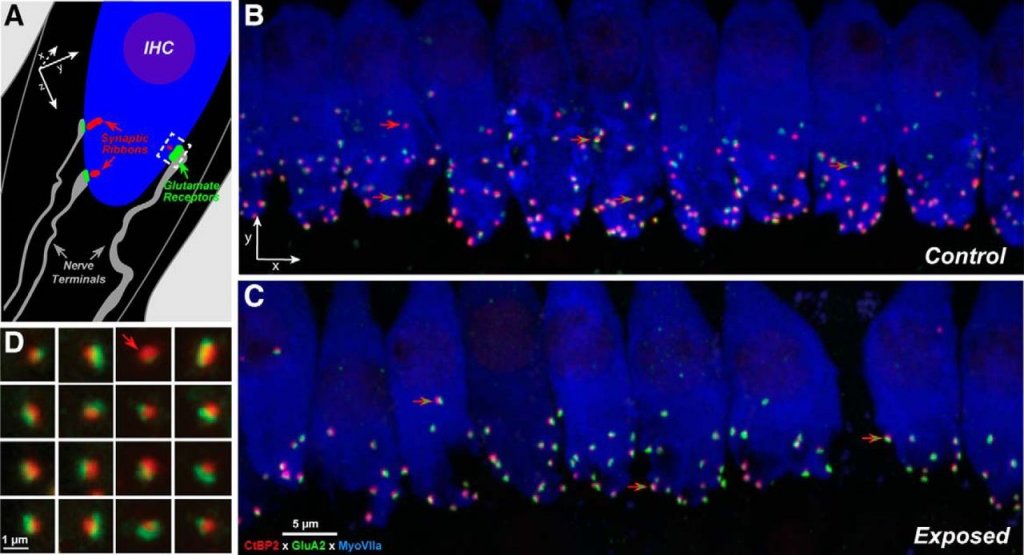
A key detail of this cochlear synaptopathy was that the synapses and nerve fibres most affected by noise exposure were those with the physiological property of a high threshold for firing; that is, the external sound levels needed to activate these synapses were above thresholds of audibility (i.e., the suprathreshold).17 On the other hand, low-threshold synapses, those likely associated with thresholds of audibility and thus the audiogram, were less affected. Put more simply, moderate noise exposure that does not elevate thresholds is damaging to synapses and nerve fibres in the inner ear that are adept at encoding features of sounds at moderate to high listening levels, but less so at low levels.
What has followed is more than 10 years of devoted research on cochlear synaptopathy, testing the hypothesis that this pathology in part explains why individuals with normal audiograms (intact low-threshold synapses) have listening difficulties under circumstances where there is background noise or multiple talkers (a loss of high-threshold synapses), as well as the emergence of “hyperactive” auditory disorders of hyperacusis and tinnitus.18
No Established Synaptopathy Tests
Before addressing the search for synaptopathy in tinnitus, it is important to highlight that at the time of this writing, there are no established physiological or behavioural tests that indicate the presence of synaptopathy in humans, and there are numerous challenges that stand in the way of such an assay. (For a thorough review, see Bramhall et al.19) So far, confirmation of synaptopathy in humans has come only from the imaging of temporal bones in cadavers. Experimentally, a major challenge for human research (versus animal research) is that to isolate the effects of synaptopathy, a study must tightly control for inter-individual factors, such as chronological age and biological sex, and other forms of damage to the ear, such as general hair cell and middle ear dysfunction. It is hard to find adults who have had noise exposure to a degree sufficient for synaptopathy but who do not have hair cell damage that may elevate thresholds and confound measurement. Further, it is difficult to determine how much mild to moderate noise exposure a person has experienced in their lifetime, and for a person to accurately remember episodes of noise exposure (but see the article by Guest et al.20). Thus, the search for a link between synaptopathy and tinnitus has become intertwined with the search for a robust measure of synaptopathy in humans, and a lesson in implementing the proper controls to rule out non-synaptopathic factors that affect physiological and behavioural measures.
The remainder of this section focuses on three candidate physiological measures of cochlear synaptopathy: suprathreshold auditory brainstem response (ABR) wave I responses, envelope following responses (EFRs), and the middle ear muscle reflex (MEMR). It is possible to argue that physiological tests provide an advantage over behavioural tests because the variability within behavioural tests is associated with higher-level factors such as cognition and downstream sensory processing ability, which are distanced from the status of synapses in the cochlea. (An exception to this might be the measurement of extended high-frequency audiometric threshold shifts; however, these shifts and synaptopathy would co-occur and not directly reflect the degree of synapse loss.)
Suprathreshold ABR Wave I Responses
Wave I of the ABR is a scalp-recorded electrical potential evoked by clicks or brief tones, and it reflects the synchronous firing of the auditory nerve a few milliseconds following the onset of stimulus. In principle, ABR wave I is an ideal synaptopathy measure because its generator occurs proximal to the lesion site. Consistent with the idea of damage to high-threshold synapses, evidence of synaptopathy appears as a reduction in wave I evoked at suprathreshold levels (typically above 80 dB SPL[sound pressure level] in humans). So far, this signature has been a reliable indicator of synaptopathy in animal models.
A paper published by Roland Schaette and David McAlpine21 was one of the first to report that individuals with tinnitus had a reduction in the suprathreshold wave I amplitude compared with people who did not have tinnitus (“non-tinnitus controls”). (Coincidentally, it was this paper that coined the term “hidden hearing loss,” which is often synonymous with “cochlear synaptopathy.”) However, since the Schaette and McAlpine paper, several studies (such as a review by Milloy et al.22) have found only mixed evidence that there are reductions in wave I amplitudes in cases of tinnitus with a normal audiogram. Thus, we are left without a conclusive answer as to whether the ABR can identify synaptopathy in people with tinnitus.
These equivocal findings may be a consequence of several factors: ABR wave I may not be as sensitive to synaptopathy in humans as it is in animals; the technical setup of these studies may have differed too widely or not controlled extraneous variables between tinnitus and control groups (e.g., age, gender, audiograms); or synaptopathy might not explain all cases of tinnitus and normal thresholds. A complicating factor is that wave I amplitude appears to receive most but not all of its contributions from low-threshold nerve fibres,23 whereas high-threshold fibres are those most affected by noise exposure. Recent attempts (for example, by Harris et al.24) have used other features of the wave I, such as amplitude growth as a function of click level and area under the wave I response to better image the contribution of high-threshold fibres.
Envelope Following Responses
TheEFR is an electrical potential measured similarly to ABRs, but it reflects a response that is phase locked to an amplitude-modulated sound. For example, the presentation of a pure tone that is amplitude modulated by 80 Hz evokes a measurable scalp-recorded neural oscillation also at 80 Hz (Figure 3). For amplitude modulation rates at 80 Hz and above, the response originates from several peripheral and brainstem regions, including the auditory nerve and midbrain. Several authors have raised the idea that EFRs are a desirable synaptopathy measure, under the rationale that this measure better reflects the contribution of high-threshold nerve fibres (those targeted by noise exposure) owing to the superiority of these fibres to track envelope modulations.25,26
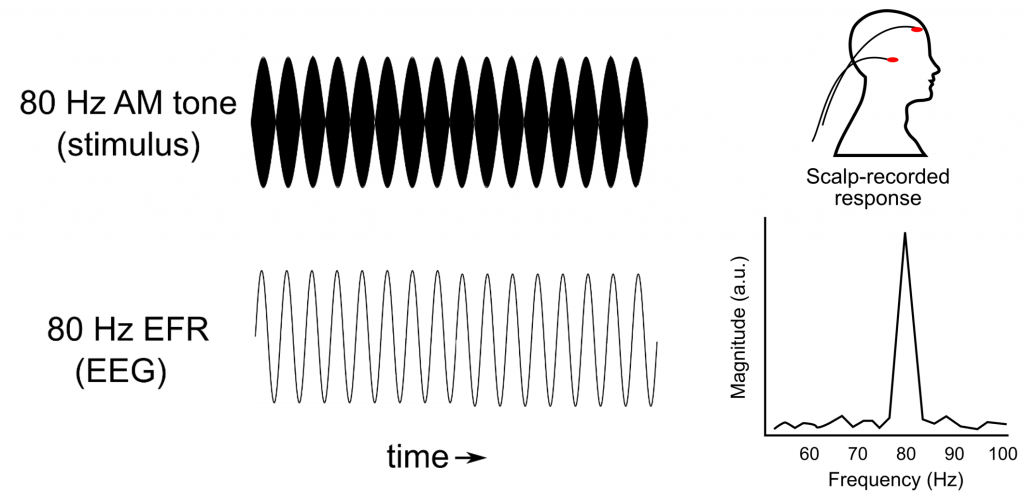
Compared with studies of ABRs, fewer EFR studies in tinnitus have been conducted. Two reports have found that EFRs of individuals with tinnitus do appear to be lower in magnitude compared with those in non-tinnitus controls, but this difference was not statistically significant.9,27,28 Thus, we are left with the same conclusion we reached with ABR wave I responses: it is unclear if EFR reductions are sensitive to detect hidden synaptic pathology in tinnitus, if present. Nonetheless, because so few data are available, there may be a potential to refine EFRs as a viable diagnostic tool. Furthermore, recent data suggest that certain stimulus parameters used to evoke EFRs may help to uncover synaptopathy in the presence of additional hair cell damage,29 which is usually a confounding variable. These advancements are promising for the majority of tinnitus cases where threshold shifts are present.
Middle Ear Muscle Reflex
The MEMR operates to protect the ear from loud noise damage by contracting the stapedius and tensor tympani muscles. The MEMR appears to be driven by the activity of high-threshold synapses and nerve fibres, which constitute a circuit that involves the cochlear nucleus and motor neurons that control middle ear muscles. The rationale for MEMR measurement is that if high-threshold synapses are lost, then the circuit is broken, and the MEMR strength measured from the ear canal should be reduced.30
In agreement with the existence of an association between tinnitus and synaptopathy, Wojtczak and colleagues31 reported that individuals with tinnitus and a normal audiogram had considerably weaker MEMRs with increasingly intense wideband click elicitors compared with non-tinnitus controls (Figure 4). As with ABRs and EFRs, however, not all MEMR findings are in broad agreement. A MEMR study conducted recently by Guest et al.32 in a younger sample of adults than those in the report by Wojtczak et al. and with widely different measures found no difference in MEMR thresholds between people who had tinnitus and those who didn’t. (Contrast this to the MEMR strength above threshold reported by Wojtczak et al.31) Direct or conceptual replications of these experiments have not yet been reported. Similar to the EFR, the use of the MEMR to detect synaptopathy is promising because of its ease of measurement compared with evoked potentials,33 and more studies in people with tinnitus are needed to fully understand MEMR’s utility.
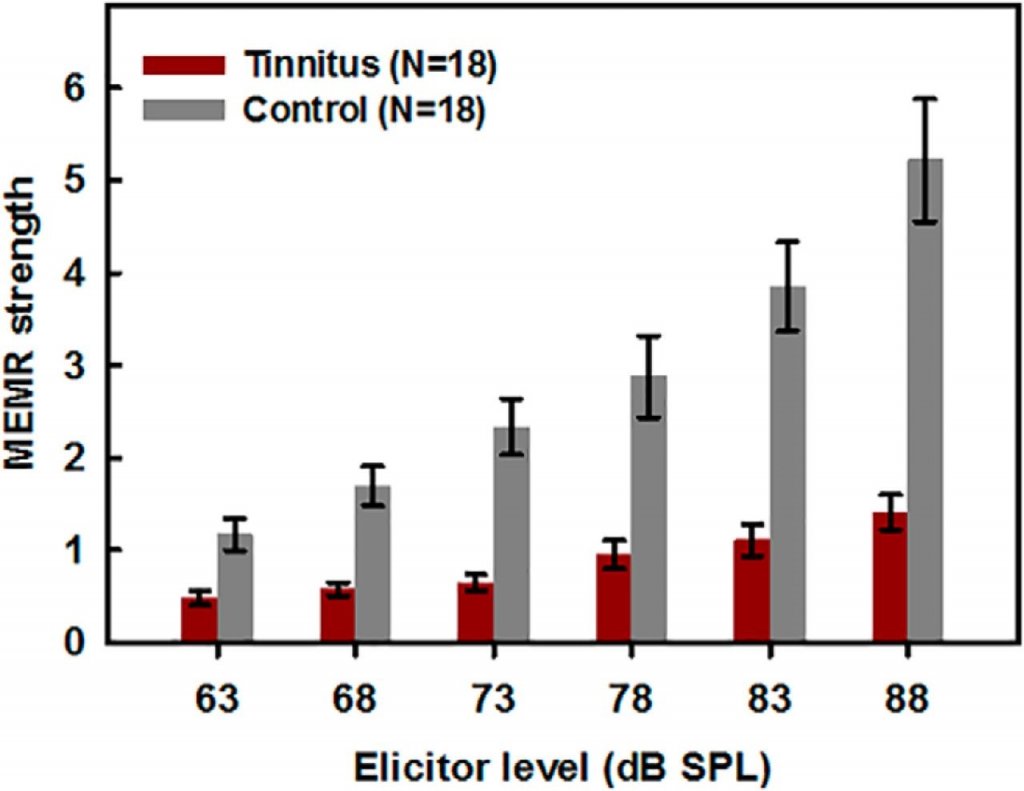
Concluding Remarks
The above studies illustrate that there are many ways that hearing damage can evade the conventional audiogram. However, there are no clear answers to (1) whether the majority of tinnitus cases with normal thresholds are associated by an obscured form of hearing damage, and (2) whether there is a battery of tests beyond the audiogram that can be used to robustly identify patterns of hearing damage related to tinnitus. This is a major roadblock on the path of tinnitus treatment.
A way to move forward is to advocate for more research. There is not yet enough careful science to either suggest or rule out candidate tests of hearing function related to tinnitus, especially those discussed for synaptopathy such as the ABR, EFR, and MEMR. We must establish conventions for physiological testing (devices and signal processing) and adopt them internationally; conduct additional thoughtful experiments; implement tighter controls (age, biological sex, occupation); and because the effects of hearing damage on physiological function are likely small, drastically increase the sample sizes of studies. No matter the outcome, at a minimum, patients with tinnitus will likely require assessment beyond the conventional audiogram for clinicians to better understand the status of the ear.
Acknowledgements
I thank Ian Bruce for his helpful comments and edits on the final version of this article.
References
- Roberts LE, Eggermont JJ, Caspary DM, et al. Ringing ears: the neuroscience of tinnitus. J Neurosci 2010 Nov 10;30(45):14972–9.
- Roberts LE. Neural plasticity and its initiating conditions in tinnitus. HNO 2018 Mar 1;66(3):172–8.
- Husain FT. Neural networks of tinnitus in humans: elucidating severity and habituation. Hear Res 2016 Apr 1;334:37–48.
- Henry JA, Dennis KC, Schechter MA. General review of tinnitus. J Speech Lang Hear Res 2005 Oct;48(5):1204–35.
- Barnea G, Attias J, Gold S, Shahar A. Tinnitus with normal hearing sensitivity: extended high-frequency audiometry and auditory-nerve brain-stem-evoked responses. Audiology 1990 Jan 1;29(1):36–45.
- Carhart R, Tillman TW. Interaction of competing speech signals with hearing losses. Arch Otolaryngol 1970 Mar 1;91(3):273–9.
- Xiong B, Liu Z, Liu Q, et al. Missed hearing loss in tinnitus patients with normal audiograms. Hear Res 2019 Dec 1;384:107826.
- Vielsmeier V, Lehner A, Strutz J, et al. The relevance of the high frequency audiometry in tinnitus patients with normal hearing in conventional pure-tone audiometry. BioMed Res Int 2015 Oct 25;2015:302515.
- Paul BT, Bruce IC, Roberts LE. Evidence that hidden hearing loss underlies amplitude modulation encoding deficits in individuals with and without tinnitus. Hearing Res 2017 Feb 1;344:170–82.
- Lobarinas E, Salvi R, Ding D. Insensitivity of the audiogram to carboplatin induced inner hair cell loss in chinchillas. Hearing Res 2013 Aug 1;302:113–20.
- Weisz N, Hartmann T, Dohrmann K, et al. High-frequency tinnitus without hearing loss does not mean absence of deafferentation. Hearing Res 2006 Dec 1;222(1–2):108–14.
- Gilles A, De Ridder D, Van de Heyning P. No cochlear dead regions detected in non-pulsatile tinnitus patients: an assessment with the threshold equalizing noise (sound pressure level) test. Noise Health 2013 May 1;15(63):129.
- Thabet EM. Evaluation of tinnitus patients with normal hearing sensitivity using TEOAEs and TEN test. Auris Nasus Larynx. 2009 Dec 1;36(6):633–6.
- Gouveris H, Maurer J, Mann W. DPOAE-grams in patients with acute tonal tinnitus. Otolaryngol Head Neck Surg 2005 Apr;132(4):550–3.
- Kujawa SG, Liberman MC. Synaptopathy in the noise-exposed and aging cochlea: primary neural degeneration in acquired sensorineural hearing loss. Hearing Res 2015 Dec 1;330:191–9.
- Fernandez KA, Jeffers PW, Lall K, et al. Aging after noise exposure: acceleration of cochlear synaptopathy in “recovered” ears. J Neurosci 2015 May 13;35(19):7509–20.
- Furman AC, Kujawa SG, Liberman MC. Noise-induced cochlear neuropathy is selective for fibers with low spontaneous rates. J Neurophysiol 2013 Aug; 110(3):577–86.
- Roberts LE, Salvi R. Overview: hearing loss, tinnitus, hyperacusis, and the role of central gain. Neuroscience. 2019 May 21;407:1.
- Bramhall N, Beach EF, Epp B, et al. The search for noise-induced cochlear synaptopathy in humans: mission impossible? Hearing Res 2019 Jun 1;377:88–103.
- Guest H, Dewey RS, Plack CJ, et al. The noise exposure structured interview (NESI): an instrument for the comprehensive estimation of lifetime noise exposure. Trends Hear 2018;22:2331216518803213.
- Schaette R, McAlpine D. Tinnitus with a normal audiogram: physiological evidence for hidden hearing loss and computational model. J Neurosci 2011 Sep 21;31(38):13452–7.
- Milloy V, Fournier P, Benoit D, et al. Auditory brainstem responses in tinnitus: a review of who, how, and what? Front Aging Neurosci 2017 Jul 21;9:237.
- Bourien J, Tang Y, Batrel C, et al. Contribution of auditory nerve fibers to compound action potential of the auditory nerve. J Neurophysiol 2014 Sep 1;112(5):1025–39.
- Harris KC, Vaden KI Jr, McClaskey CM, et al. Complementary metrics of human auditory nerve function derived from compound action potentials. J Neurophysiol 2018 Mar 1;119(3):1019–28.
- Bharadwaj HM, Verhulst S, Shaheen L, et al. Cochlear neuropathy and the coding of supra-threshold sound. Front Syst Neurosci 2014 Feb 21;8:26.
- Shaheen LA, Valero MD, Liberman MC. Towards a diagnosis of cochlear neuropathy with envelope following responses. J Assoc Res Otolaryngol 2015 Dec 1;16(6):727–45.
- Roberts LE, Paul BT, Bruce IC. Erratum and comment: envelope following responses in normal hearing and in tinnitus. Hearing Res 2018 Apr;361:157.
- Guest H, Munro KJ, Prendergast G, et al. Tinnitus with a normal audiogram: relation to noise exposure but no evidence for cochlear synaptopathy. Hearing Res 2017 Feb 1;344:265–74.
- Keshishzadeh S, Garrett M, Vasilkov V, Verhulst S. The derived-band envelope following response and its sensitivity to sensorineural hearing deficits. Hearing Res 2020 Apr 29:107979.
- Valero MD, Hancock KE, Liberman MC. The middle ear muscle reflex in the diagnosis of cochlear neuropathy. Hearing Res 2016 Feb 1;332:29–38.
- Wojtczak M, Beim JA, Oxenham AJ. Weak middle-ear-muscle reflex in humans with noise-induced tinnitus and normal hearing may reflect cochlear synaptopathy. eNeuro. 2017 Nov;4(6):ENEURO.0363-17.2017.
- Guest H, Munro KJ, Plack CJ. Acoustic middle-ear-muscle-reflex thresholds in humans with normal audiograms: no relations to tinnitus, speech perception in noise, or noise exposure. Neuroscience. 2019 May 21;407:75–82.
- Bharadwaj HM, Mai AR, Simpson JM, et al. Non-invasive assays of cochlear synaptopathy–candidates and considerations. Neuroscience 2019 May 21;407:53–66.