An Evolving Understanding of Cochlear Synaptopathy
Clues for why “hidden hearing loss” remains hidden in clinical testing
Cochlear synaptopathy1 describes the loss of synapses, important neural junctions, that connect cochlear inner hair cells to the auditory nerve. Like many sensorineural hearing pathologies synaptopathy is caused by noise exposure, aging, and toxic drugs. When cochlear synapses are destroyed, the auditory nerve fibers to which they connect degenerate, limiting the sound information carried from the ear to the brain. Cochlear synapses are more sensitive to noise exposure and aging than hair cells or their stereocilia and loss of synapses does not appear to appreciably elevate auditory thresholds. For this reason, synaptopathy is hidden from the audiogram and other standard clinical tests.
Cochlear synaptopathy is a familiar term to readers of Canadian Audiologist. Contributors have written about the challenge of identifying synaptopathy in clinical settings and what it means for rehabilitation,2,3 how researchers are using electrophysiology to find synaptopathy in humans,4,5 and whether synaptopathy can explain cases of tinnitus without measurable shifts in the pure tone audiogram.6 In the previous issue, Stéphane Maison described a word recognition test for auditory nerve degeneration after synaptic loss. A theme running through most of these articles and in the scientific literature is that synaptopathy is easy to detect and characterize in animals but difficult to identify in living humans. Studies have not found compelling evidence of synaptopathy using behavioural and physiological tests that could be modified for the clinic, like sound-in-noise perception and the ABR (see Bramhall et al.7 for a longer review). This is puzzling because synaptopathy does occur in humans and advances with age, as shown by post-mortem examination of temporal bones.8 Human research has less control over noise exposure history and other hearing variables compared to animal research, which could be one source of disagreement. In any case, the lack of consistent findings leaves some researchers doubting whether synaptopathy has clinically meaningful effects on hearing ability, at least in younger adults.
Recent research, however, paints a more complex picture of synaptopathy. It may differ from species to species and affects the auditory nerve in paradoxical ways. This work may explain why synaptopathy remains elusive in human testing. Here, I briefly review this work and its implications for clinical research.
Cochlear Synapses Can Regenerate Following Noise Exposure
The original research by Kujawa and Liberman9 used mice in their studies and found that synaptopathy was permanent. A mild noise exposure (100 dB SPL octave-band noise for two hours) produced a ~50% loss of cochlear synapses that never recovered, while hair cells and hearing thresholds remained unaffected. The mouse strain used in this study and other synaptopathy experiments (the CBA/CaJ strain) was known to have less hearing decline as it matures, allowing researchers a cleaner look at noise exposure effects outside of aging.
Experiments in guinea pigs, however, suggest that synapses may regenerate over time, even in adulthood. Initially reported by Shi and colleagues in 201310, Hickman et al.11,12 followed up and found that an 80% loss of synapses recovered to within 10% of the pre-exposure numbers within six weeks. The recovery of guinea pig synapses following noise exposure is shown in Figure 1 below and are compared to the mouse. Cochlear synapses are clustered toward the base or bottom of the hair cell (Figure 1A). One week after noise exposure in the guinea pig, nerve fibers appear to regenerate or migrate to positions higher up the hair cell towards its apex (the top; Figure 1B), which eventually return back to the base of the hair cell one month after noise exposure (Figure 1C). In the mouse (bottom row), synaptopathy is apparent one week after exposure with no similar evidence of nerve fiber regeneration after one week (Figure 1E). Interestingly, synapses in the mouse do recover if the experimenters apply the drug NT-3, a neurotrophic factor that promotes axon growth and neuronal survival13 (Figure 1F). Synapse regeneration is not specific to guinea pigs but also occurs in another mouse strain (C57BL/6)14.
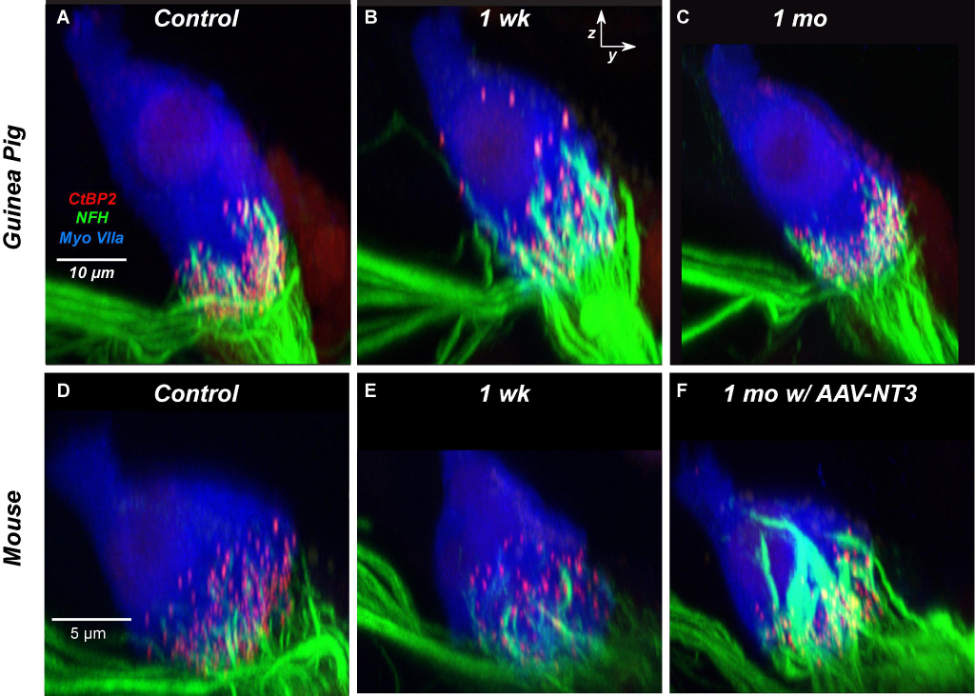
Synapse regeneration in the guinea pig and some mice types, but not the CBA/CaJ mouse, suggests the existence of inter-species or inter-strain differences in how cochlear synaptopathy unfolds. Although humans show evidence of synaptopathy in post-mortem inspection of temporal bones,8 an interesting question is whether synaptic regeneration occurs in humans and to what extent. Until verified by research studies, we cannot assume that a single noise exposure necessarily leads to irreversible synapse loss in all mammals like in CBA/CaJ mice.
Understanding species-related differences in synaptopathy is essential for designing drugs that help repair synaptic connections, an intervention that may help with age-related difficulty in hearing and speech perception.15 Synapse regeneration may also explain the frustrating search for synaptopathy in humans. Effects of noise exposure on young adults’ synapses may not appear in behavioural or electrophysiological assays until enough damage accumulates over the lifespan,14 stressing the need for assays that can “see” synapse loss in living humans among the expected decline of hair cells and other age-related changes to the inner ear16.
Synaptopathy Affects All Auditory Nerve Fiber Types and May Result In Improved Nerve Responses
Early studies on synaptopathy found that mild noise exposure targeted a vulnerable subset of the synapses found on inner hair cells – those which connect to nerve fibers that are important for encoding acoustic features at medium/high sound levels and in background noise.17 These findings were provocative because if synaptopathy did not affect hearing thresholds or hair cell function but did affect synapses and nerve fibers that helped with everyday listening in noise, it could explain why some people have hearing difficulty despite normal audiograms. The vulnerable fibers are called low-spontaneous rate fibers, or low-SR fibers, referring to the low rate at which these nerves fire action potentials without external stimulation. Inner hair cells are also connected to high-SR fibers, sensitive to lower sound levels like those used in audiometry but more resistant to noise exposure than low-SR fibers.
Hickman et al.13 confirmed that synaptic loss most strongly affected the vulnerable low-SR fibers compared to high-SR fibers in the guinea pig. However, another species difference arose. Suthakar and Liberman18 examined how low- and high-SR fibers were affected in the CBA/CaJ mouse more closely. They found a comparable loss of both high- and low-SR fibers, not just low-SR fibers. Another recent study found a mixture of high-SR and low-SR fiber loss in the gerbil, another animal used to study synapse loss.19 If high-SR fibers are also affected by synaptopathic noise exposure, what happens to in sound encoding in the auditory nerve? To find out, Suthakar and Liberman went a step further – they examined physiological responses of single auditory nerve fibers after noise exposure. Surprisingly, surviving nerve fibers were more excitable and improved in function, contrary to expectations. They had larger responses to the onset of sound, the response timing was more consistent, and they were more resistant to the presence of background noise. It is not clear why the auditory nerve changes in this way after synaptopathic noise exposure, and only new experiments can offer an answer.
The work by Suthakar and Liberman may have interesting implications for human research. If synaptopathy affects all nerve fiber types and leads to a gain in the function of surviving nerve fibers, human researchers must reconsider their approach. Tests looking for sound encoding deficits might not work well if synapse loss results in sound encoding improvements. Mix in other messy factors about human hearing studies, such as limited knowledge of noise exposure and drug history, potential genetic factors, and other individual differences,7 then maybe the difficulty of reconciling human and animal research is not so surprising.
Concluding Remarks
Cochlear synaptopathy persists as a hot topic in hearing research and auditory neuroscience, and there is intense debate on developing a clinical test for it. Unfortunately, the search for synaptopathy in living humans using behavioural and physiological methods has been far less successful than efforts made in animal models of hearing. However, our understanding of synaptopathy is evolving. Less than a decade ago, synaptopathy was viewed as irreversible and concentrated on nerve fibers (low-SR types) that encode sounds at moderate to high levels and in background noise. This made sense if synapse loss was behind listening-in-noise problems in adults with normal audiograms. However, we have evidence that cochlear synaptopathy recovers in some species, may implicate both high- and low-SR fiber types, and is accompanied by paradoxical improvements in auditory nerve fibers’ sound encoding ability. It remains to be seen whether these results apply to human research, but if so, we may be one step closer to developing a viable clinical test.
References
- Liberman MC, Kujawa SG. Cochlear synaptopathy in acquired sensorineural hearing loss: Manifestations and mechanisms. Hearing research. 2017 Jun 1;349:138-47.
- Le Prell CG. Hidden versus not-so-hidden hearing loss. Canadian Audiologist. 2018;5(2).
- Tremblay K. You Call it CAPD, I Call it Synaptopathy: What’s a Clinician to Do with This Information? Canadian Audiologist. 2017;4(3)
- Pienkowski M. Science Matters: Towards a Differential Diagnosis of Cochlear Synaptopathy as a Contributor to Sensorineural Hearing Loss. Canadian Audiologist. 2018;5(3).
- Sheppard A. The “No Longer Hidden” Hearing Loss and Audiology: Bridging the Research-Clinic Gap. Canadian Audiologist. 2017;4(6).
- Paul BT. Tinnitus with a Normal Audiogram. Canadian Audiologist. 2020;7(6).
- Bramhall N, Beach EF, Epp B, Le Prell CG, Lopez-Poveda EA, Plack CJ, Schaette R, Verhulst S, Canlon B. The search for noise-induced cochlear synaptopathy in humans: Mission impossible?. Hearing research. 2019 Jun 1;377:88-103.
- Wu PZ, Liberman LD, Bennett K, De Gruttola V, O’malley JT, Liberman MC. Primary neural degeneration in the human cochlea: evidence for hidden hearing loss in the aging ear. Neuroscience. 2019 May 21;407:8-20.
- Kujawa SG, Liberman MC. Adding insult to injury: cochlear nerve degeneration after “temporary” noise-induced hearing loss. Journal of Neuroscience. 2009 Nov 11;29(45):14077-85.
- Shi L, Liu L, He T, Guo X, Yu Z, Yin S, Wang J. Ribbon synapse plasticity in the cochleae of Guinea pigs after noise-induced silent damage. PloS one. 2013 Dec 9;8(12):e81566.
- Hickman TT, Hashimoto K, Liberman LD, Liberman MC. Synaptic migration and reorganization after noise exposure suggests regeneration in a mature mammalian cochlea. Scientific reports. 2020 Nov 17;10(1):1-4.
- Hickman TT, Hashimoto K, Liberman LD, Liberman MC. Cochlear synaptic degeneration and regeneration after noise: effects of age and neuronal subgroup. Frontiers in Cellular Neuroscience. 2021;15.
- Suzuki J, Corfas G, Liberman MC. Round-window delivery of neurotrophin 3 regenerates cochlear synapses after acoustic overexposure. Scientific reports. 2016 Apr 25;6(1):1-1.
- Luo Y, Qu T, Song Q, Qi Y, Yu S, Gong S, Liu K, Jiang X. Repeated moderate sound exposure causes accumulated trauma to cochlear ribbon synapses in mice. Neuroscience. 2020 Mar 1;429:173-84.
- Kujawa SG, Liberman MC. Translating animal models to human therapeutics in noise-induced and age-related hearing loss. Hearing research. 2019 Jun 1;377:44-52.
- Vasilkov V, Garrett M, Mauermann M, Verhulst S. Enhancing the sensitivity of the envelope-following response for cochlear synaptopathy screening in humans: The role of stimulus envelope. Hearing Research. 2021 Feb 1;400:108132.
- Bharadwaj HM, Verhulst S, Shaheen L, Liberman MC, Shinn-Cunningham BG. Cochlear neuropathy and the coding of supra-threshold sound. Frontiers in systems neuroscience. 2014 Feb 21;8:26.
- Suthakar K, Liberman MC. Auditory-nerve responses in mice with noise-induced cochlear synaptopathy. Journal of neurophysiology. 2021 Dec 1;126(6):2027-38.
- Jeffers PW, Bourien J, Diuba A, Puel JL, Kujawa SG. Noise-induced hearing loss in gerbil: round window assays of synapse loss. Frontiers in Cellular Neuroscience. 2021:286.