A Proposal to Standardize Gaze during oVEMP Testing using a Chin Rest
Michael Vekasi, AuD, R.Aud, Aud(C), FAAA and Erica Zaia are coordinating the “Striking the Right Balance,” column which will cover the latest information on “all things vestibular.”
In this edition of “Striking the Right Balance,” Janine Verge, audiologist with the Nova Scotia Hearing and Speech Centres and Anton Charko, third year audiology student at Dalhousie University, propose a way of standardizing gaze during Ocular VEMP testing using a chin rest.
If you are a health care professional and would like to be more involved in all things vestibular, please sign up for the Vestibular Special Interest Group. Sign up by simply emailing JanineAllison.Verge@nshealth.ca to let us know you want to be a part. Also, check out our Facebook page for a free list of on-line vestibular resources at the CAA National Vestibular Special Interest Group page.
Ocular vestibular evoked myogenic potential testing (oVEMP) is a new test used to assess otolith function. The otolith organs are comprised of the utricle and the saccule which are sensitive to gravity and linear acceleration. Information from the utricle stimulates the six ocular muscles. Utricular excitatory inputs project to the ipsilateral superior oblique, superior rectus, and medial rectus eye muscles and relaxation of the contralateral inferior oblique, inferior rectus, and lateral rectus eye muscles.1
oVEMPs are short-latency myogenic potentials produced by sound stimulation and registered through ocular myogenic responses recorded beneath the eye contralateral to the stimulated ear with surface electrodes. Sound stimulation can produce VEMPs because otoliths are sensitive to sound in amniotes (reptiles, birds, and mammals) that have been retained in humans.2 The oVEMP evaluates the ascending vestibular pathway via the vestibulo-ocular reflex (VOR). The VOR is responsible for stabilizing vision on the retina during head and body movements. There is some evidence that oVEMPs originate predominately in the utricle.3−6 whereas other findings argue the response may include a small contribution from the saccule, originating from both the utricle and the saccule.7,8
The ocular VEMP allows clinicians to effectively evaluate unilateral utricular function with a more cost effective method compared to unilateral centrifugation which relies on expensive equipment. Also, compared to the subjective visual vertical/horizontal testing, oVEMP testing is superior because results remain abnormal even after central compensation has occurred.9
The air conducted cervical VEMP (cVEMP) was first described more than 20 years ago,10 and has since been widely used to evaluate saccular function. Ocular VEMPs are an important addition to the vestibular test battery and complimentary to cervical VEMPs. They may be more sensitive than cVEMPs in the evaluation of superior semicircular canal dehiscence11 and assess the superior versus the inferior vestibular nerve.12 It is important to measure otolith function because it can help aid diagnosis, test the extent of a lesion, monitor disease progression or recovery over time, and injury may contribute to a source of disability.13 Acute injuries to the utricule may cause a subjective tilt sensation, imbalance, and visual disturbance. Enlarged oVEMPs have been shown to be sensitive for screening of superior canal dehiscence.14 Absent oVEMPs are suggestive of a loss of superior vestibular nerve otolith function which can be seen in conditions such as vestibular neuritis or vestibular schwannoma.15
The o-VEMP tracing consists of a biphasic waveform. The first peak has a negative deflection (N1) with a mean latency of approximately 10 milliseconds followed by a positive peak (P1) with a mean latency of 15 milliseconds. Since the oVEMP relies on the activation of the contralateral inferior oblique muscle, the control of muscle contraction is important for reproducible and reliable results. The inferior oblique muscle is activated when looking up, and consequently, vertical gaze elevation is more influential than horizontal gaze, head rotation, body position, or vision on oVEMP amplitude and latency.16 Even increases in vertical gaze as small as 5 degrees produce significant changes in amplitude.17 This effect is thought to be related to changes in both tonic contraction and displacement of the inferior oblique muscle.18 Instructing a patient to maintain his or her maximum vertical gaze is not enough: not only is maximum vertical gaze subjective, but the ability to stretch your gaze in any one direction varies significantly as a function of age. While younger adults are able to stretch their gaze to about 50 to 60 degrees in any direction, older adults have a reduced range of eye movement,19 with a marked decline beginning in the sixth decade of life. Shechtman et al. measured maximum ocular duction in 204 healthy volunteers and found that, compared to their younger counterparts, older adults aged 80 to 95 were able to look about 25% less far down and to the left and right.19 Interestingly, the greatest reduction was measured when looking up, where the average decline was 35% in the same group. This finding is consistent with other studies of voluntary eye movement, which found upward gaze to be the most affected by age related deterioration.20,21
In clinical practice, the use of 30 and 35 degree upward gaze angles are most commonly used. Some clinicians vaguely ask patients to maximally look upward to elicit the oVEMP. Kantner and Gürkov report maximal upward gaze is quite subjective and differs among subjects, which impedes comparisons of oVEMP results.17 Therefore, maximal upward gaze does not permit controlling the level of gaze to avoid confounding effects by different gaze angles. Based on their research examining the effects of commonly used upward gaze angles on oVEMPs, they recommend using the 35-degree upward gaze angle for recording oVEMP. However, since testing can be tiring at 35 degrees and elderly persons might not be able to perform such an elevation of the eye, the 30-degree angle may also be considered as sufficient, as long as the maintenance of exactly the same degree of gaze elevation is accurately controlled.22
Standardizing gaze is important because voluntary or involuntary changes in gaze elevation between subjects or between trials in the same subject may make interindividual or intraindividual comparison of results inappropriate and may have an effect on normative data. Also, VEMP testing plays an important role in SSCD diagnosis which relies on amplitude measures of the N1-P1 response. Different levels of gaze when monaurally recording oVEMP may confound the effects of comparing a patient‘s left and right oVEMP and may lead to miscalculation of asymmetry ratios. Thus, it is crucial that gaze is controlled for when administering oVEMPs. Although there are other variables to consider when performing ocular VEMPs such as electrode positioning23 and stimulus repetition rate,24 the focus of this article is on proposing a simple standardized method to control for gaze during oVEMP testing.
The following are three different methods reported to attempt to standardize gaze:
- Jerin et al reported a need to obtain accurate standardization of gaze when recording oVEMPs and suggest optimal oVEMP reproducibility can be achieved when using a visual target which ensures a constant upward gaze angle without modification by the subject’s head movements.25 They examined the use of a head mounted laser to produce a visual target to enhance the reproducibility of oVEMP results. They report the laser headband ensures a constant gaze angle and rules out the influence of small involuntary head movements on the gaze angle. When looking upward at a target fixed on the wall, small head movements—whilst the gaze is correctly fixated on the target—change the relative position of the eyeball within the orbit, which in turn alters the gaze angle. In this way, both neck flexion and neck extension might occur, which might impair the reproducibility without systematically affecting the absolute amplitudes. They also note that with the help of the head-mounted laser pointer, the gaze angle remains constant even when the subject moves his head ago.25
Possible Limitations: The laser headband is not a product currently available for sale for clinicians. It is unclear whether the laser pointer fixed on the forehead with a constant upward gaze angle of 35 degrees took into account the variable differences in distance between the laser projection point and the eye level of each subject. Also, the authors report there might have been issues other than head movement which influenced the results such as luminosity of the laser target. They reported that the absolute amplitudes were on average slightly larger in the wall target group they compared to the laser headband. - Murnane et al examined the effects of stimulus and recording parameters on the air conduction ocular vestibular evoked myogenic potential.26 To standardize gaze, the subjects in this study were positioned supine on an examination table in a dimly lit sound treated booth and instructed to direct their gaze at a visual target projected on the ceiling at a distance of 1 m at a neutral (0°) angle. A weighted plumb line was suspended from the neutral target on the ceiling, and each subject's head location was adjusted to ensure that the target was at 0° and at midline. The subjects were instructed to direct their gaze at a visual target at a vertical elevation of 30° and oVEMPs were then obtained ago.26
Possible Limitations: This method does not account for head movement during testing as the neutral target was only established at the beginning of testing. It remains unclear how the plumb line was used and if any intersubject variability in head angle in the supine position was accounted for. The concern would be if the head was at different angles at “midline” would this alter each subjects range of gaze. - Kantner and Gürkov studied the effects of commonly used upward gaze angles on oVEMPs.17 To standardize gaze, subjects were instructed to look upward at one of the markings on the wall, which were positioned 30 and 35 degrees above eye level at a distance of approximately 80 cm, and were asked to maintain maximal upward gaze, respectively. The researchers reported the position of the eye was regularly checked by the examiner during the measurements.
Possible Limitations: This method does not account for head movement during testing. It does not explain what method was used by the examiner during regular checks to ensure the position of the eye during testing. It also does not explain how the researchers determined accurately what each subjects eye level was and how the target markings on the wall were moved accordingly.
Our proposal for standardizing gaze during oVEMP testing uses a chin rest (see below). Advantages of using a table mounted chin rest are that it is a standard piece of equipment for many clinical uses, is already available for purchase, and has a low cost. The eye level can be kept standardized by placing a marker on the side of the chin rest and moving the patients chair or chin rest accordingly to get each patients eye to the set target.
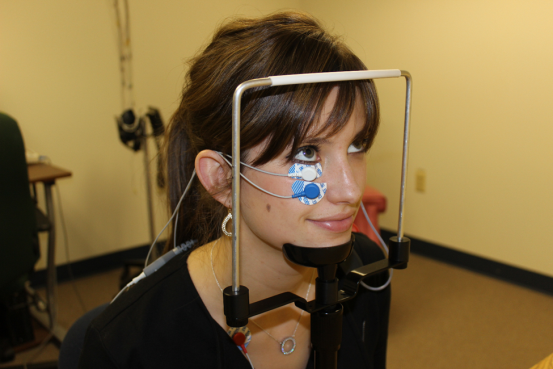
Alyson Earle: Dalhousie University Audiology Student
The gaze target must be located on a wall at least 30 degrees above eye level. To determine where to set your marker on the wall, measure the height of the eye level set target on the chin rest from the floor as well as the distance from the chin rest to the wall. For a 30o angle, the height of the gaze target is always tan (30o) or approximately 0.57735 the distance from the wall. For example, if the eye level set target is located 92 cm from the wall and 120 cm from the floor, the gaze target would be placed 53.12 cm (92 cm × 0.57735) above the eye level set target or 173.12 cm (53.12 cm + 120 cm) above the floor.
It is our recommendation that further research be conducted to determine which method is best to standardize gaze during oVEMP testing including this proposed method. Also, once finalized, a written detailed description of the methodology used would be beneficial to ensure consistency across examiners.
References
- Chihara Y, Iwasaki S, Ushio M, Murofushi T. Vestibular-evoked extraocular potentials by air-conducted sound: another clinical test for vestibular function. Clin Neurophysiol 2007;118:2745–51.
- Maruska KP and Mensinger AF. Directional sound sensitivity in utricular afferents in the toadfish Opsanus tau. J Exp Biol 2015;218(Pt 11):1759–66.
- Curthoys IS, Vulovic V, and Manzari, L. Ocular vestibular-evoked myogenic potential (oVEMP) to test utricular function: neural and oculomotor evidence. Acta Otorhinolaryngol Ital 2012;32(1):41.
- Curthoys IS. A critical review of the neurophysiological evidence underlying clinical vestibular testing using sound, vibration and galvanic stimuli. Clin Neurophysiol 2010;2(1):132–44.
- Curthoys IS, Manzari L. Evidence missed: ocular vestibular-evoked myogenic potential and cervical vestibular-evoked myogenic potential differentiate utricular from saccular function. Otolaryngol Head Neck Surg 2011;144:751–52.
- Manzari L, Burgess AM, Curthoys IS. Dissociation between cVEMP and oVEMP responses: different vestibular origins of each VEMP? Eur Arch Otorhinolaryngol 2010;267:1487–89.
- Papathanasiou ES. Ocular vestibular evoked myogenic potentials (OVEMPs): saccule or utricle? Clin Neurophysiol 2012;123:216.
- Welgampola MS, Carey JP. Waiting for the evidence: VEMP testing and the ability to differentiate utricular versus saccular function. Otolaryngol Head Neck Surg 2010;143:281–83.
- Weber KP and Rosengren SM. Clinical utility of ocular vestibular-evoked myogenic potentials (oVEMPs). Curr Neurol Neurosci Rep 2015;15:22.
- Colebatch JG, Halmagyi GM, and Skuse NF. Myogenic potentials generated by a click-evoked vestibulocollic reflex. J Neurol Neurosurg Psychiatr 1994;57(2):190–97.
- Zuniga MG, Janky KL, Nguyen KD, et al. Ocular vs. cervical VEMPs in the diagnosis of superior semicircular canal dehiscence syndrome. Otol Neurotol 2013;34(1):121.
- Curthoys IS, Iwasaki S, Chihara Y, et al. The ocular vestibular-evoked myogenic potential to air-conducted sound; probable superior vestibular nerve origin. Clin Neurophysiol: Off J Int Fed Clin Neurophysiol 2011;122(3):611–6.
- Lempert T, Gianna CC, Gresty MA, and Bronstein AM. Effect of otolith dysfunction. Brain 1997;120:1005–13.
- Piker E. Update on cVEMP and oVEMP testing in superior canal dehiscence. Can Audiol 2014;1(4): Available at: https://canadianaudiologist.ca/update-on-cvemp-and-ovemp-testing-in-superior-canal-dehiscence/.
- Ropper AH. Illusion of tilting of the visual environment: report of five cases. J. Clin Neuro-ophthalm 1983;147–51.
- Govender S, Rosengren SM, and Colebatch JG. The effect of gaze direction on the ocular vestibular evoked myogenic potential produced by air-conducted sound. Clinical Neurophysiol 2009;120(7):1386–91.
- Kantner C and Gürkov R. The effects of commonly used upward gaze angles on ocular vestibular evoked myogenic potentials. Otol Neurotol 2014;35(2):289–93.
- Rosengren SM, Colebatch JG, Straumann D, and Weber KP. Why do oVEMPs become larger when you look up? Explaining the effect of gaze elevation on the ocular vestibular evoked myogenic potential. Clin Neurophysiol 2013;124(4):785–91.
- Shechtman D, Shallo-Hoffmann J, Rumsey J, et al. Maximum angle of ocular duction during visual fixation as a function of age. Strabismus 2005;13(1):21–6.
- Chamberlain W. Restriction in upward gaze with advancing age. Am J Ophthalmol 1971;71(1):341–46.
- Clark RA and Isenberg SJ. The range of ocular movements decreases with aging. Journal of American Association for Pediatric Ophthalmol Strabismus 2001;5(1):26–30.
- Kantner C and and Gürkov R. The effects of commonly used upward gaze angles on ocular vestibular evoked myogenic potentials. Otol Neurotol 2014;35:289–93.
- Sandhu JS, George SR, and Rea PA. The effect of electrode positioning on the ocular vestibular evoked myogenic potential to air-conducted sound. Clin Neurophysiol 2013;124(6):1232–36.
- Singh NK, Kadisonga P, and Ashitha P. Optimizing stimulus repetition rate for recording ocular vestibular evoked myogenic potential elicited by air-conduction tone bursts of 500 Hz. Audiol Res 2014;4(1).
- Jerin C, Bartl K, Schneider E, and Gürkov R. Enhancing the reproducibility of ocular vestibular evoked myogenic potentials by use of a visual target originating from a head-mounted laser. Eur Arch Oto-Rhino-Laryngol 2014;1–4.
- Murnane OD, Akin FW, Kelly KJ, and Byrd S. Effects of stimulus and recording parameters on the air conduction ocular vestibular evoked myogenic potential. J Am Acad Audiol 2011;22(7):469–80.