Measuring Directionality of Modern Hearing Aids
Introduction
Directionality in hearing aids is a key factor in understanding speech in noise, especially in very complex and demanding situations. Hearing aids typically use at least two microphones with omnidirectional characteristics in order to compute directional signals, resulting in what is called a differential directional microphone. The performance of these microphones can be evaluated using sound sources from different directions (e.g., ANSI S3.35-20041), and findings such as the Directivity Index (DI) can be derived from the measurements. These measurements, however, are dependent on the assumption that the device under test has a static directional performance. Since the introduction of the adaptive directional microphone,2 this is no longer always applicable. The adaptive directional microphone was the first system to actively exploit, that in everyday listening scenarios, sound sources often are not coming from all directions at once, a fact referred to as spatio-temporal sparsity. New beamforming technologies further exploit this principle in a binaural manner, where noise suppression is not only applied to the rear hemisphere but also to interfering side speakers.3 The work of Powers and Fröhlich4 reveals that these new algorithms significantly improve speech recognition in certain background noise scenarios. Furthermore, they are thoroughly integrated with noise reduction algorithms, which makes it necessary to evaluate the entire system, more or less as a “black box” approach.
Measurement Method
Our proposed new method is based on the previous work of Hagerman and Olufsson5 and extends the approach suggested Wu and Bentler6. It is described in detail in another recent publication.7
In order to assess directionality, and relate this to real-world performance, it makes sense to use speech rather than synthetic signals. The international speech-test signal (ISTS),8 which consists of syllables of female speakers in six different languages, shaped to the international long term average speech signal (ILTASS) was chosen. To account for diffuse signal components in loud environments, pink noise was added at a much lower level. The hearing aids were fitted to the Knowles Electronics Manikin for Acoustic Research (KEMAR) and the target signal was presented from our defined target direction of 0° azimuth. The azimuth of the interfering signal changes sequentially, using eight speakers located at multiples of 45°. For each angle, the signal was played and recorded twice, using an inverted phase for the interferer signal for the second playback. Having both recordings it is then possible to reconstruct the signal components of the recorded signal originating from the target and the interfering speaker under the assumption that the device under test behaves equally for both test runs and is not altered by a hidden internal state (e.g., a classification system). The correctness of this assumption can be checked easily by playing only an interferer signal twice and calculating the difference between both measurements.
In order to increase the spatial resolution of the measurement with a limited number of speakers, KEMAR was rotated by 22.5 degrees and the measurements were repeated, as depicted in Figure 1 (left panel). The strength of this method is that not only can the influence on the interfering speaker be evaluated, but also a possible influence on the target speaker can be quantified. In order to increase the signal-to-noise ratio (SNR) for a target signal, the (logarithmic) difference between the two– which we call interferer-to-target ratio (ITR) – is most important, as it effectively resembles the inverse SNR benefit. In total, this procedure then provides a total of 16 measurements at 22.5 degree sequential azimuths surrounding the KEMAR, which can be used for assessment of directionality.
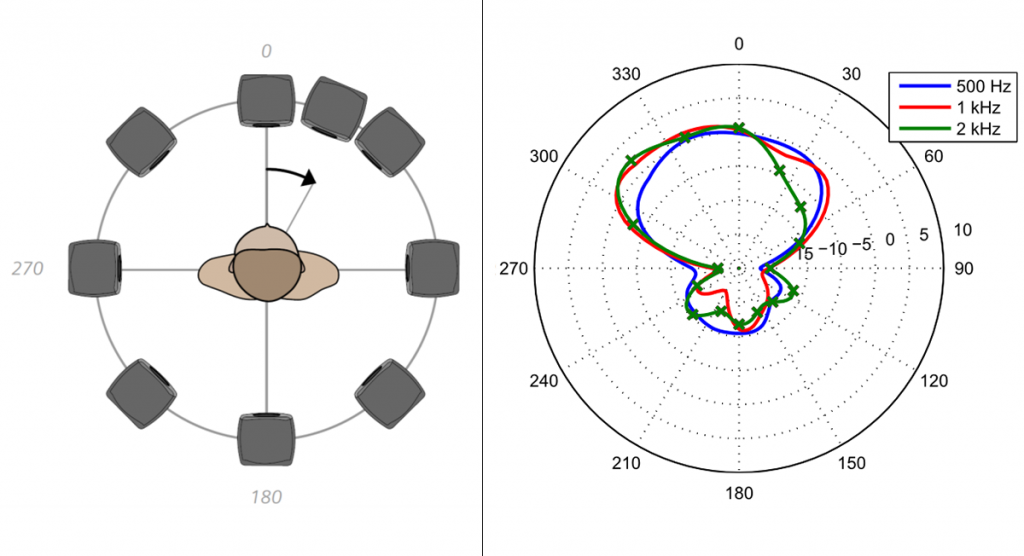
Figure 1: Left panel: Measurement setup. The KEMAR is rotated 22.5 degrees for the second round of testing. For more details about the approach, please refer to Aubreville and Petrausch7. Right panel: Interferer to Target Ratio (ITR) for standard directionality at three different frequencies.
The attenuation provided by the noise reduction scheme can be calculated over angle and frequency bands, as depicted in Figure 1 (right panel). Besides polar plots, a single figure of performance over frequency is desirable, summarizing over the entire angular range. This is also conducted for the calculation of the directivity index (DI), as described in ANSI S3.35-2004.1 The DI, however, is only defined for a static behaviour of the system under test, and adaptive technologies do make a difference for sparse spatial conditions. This is also true for the assessment of speech intelligibility.9 We propose, therefore, a calculation of a sequential directivity index (sDI), which is similar to the DI, but applied on the sequentially calculated ITR values.7 These should, as the DI, be calculated in octave bands to account for the logarithmic scaling of human hearing.
The aim of the sDI is to provide values that can facilitate comparison between different directional processing schemes. To illustrate this, measurements were made of different directional modes for devices with different form factors and performance levels in order to show the performance of each. All measurements were carried out at a mean signal level of 85 dB(A), which is a realistic condition for loud environments, a typical use case for binaural beamforming schemes.
To evaluate possible differences in two-microphone-device form factors, three styles of hearing aids were tested: 1. mini-BTE receiver-in-the-canal (RIC) devices (Siemens Pure Carat 7bx), 2. traditional behind-the-ear(BTE) devices (Siemens Motion PX 7bx and Motion SX 7bx), 3. in-the-canal (ITC) devices (Siemens Insio ITC 7bx). We also conducted testing with a fourth product, a one-microphone completely-in-the-canal (CIC) device which also utilizes binaural beamforming (Siemens Insio CIC 7bx).
Measurement Results for BTE Devices
Frequency-Dependent Behaviour
Our first device under test was the mini-BTE RIC product which was fitted to a 40 dB flat hearing loss using the proprietary Siemens binaxFit™ for experienced users, with double domes for acoustic coupling. Compression ratios were set to 1:1 to yield linear amplification, and feedback cancellation was disabled. Three different microphone modes were investigated: omnidirectional, monaural directional and binaural directional. The hearing aids were fitted to the KEMAR, and for simplicity, only the measurements obtained for the left ear are shown. For the BTE devices, the omnidirectional setting is not 100 percent omnidirectional, as it includes a pinna compensation algorithm termed TruEar™, which closely resembles the natural directionality of the external ear. As shown in Figure 2, it was found that directionality increases in general with the microphone mode. It is, however, somewhat frequency-dependent. For example, for 500Hz the binaural directivity only provides a minor advantage over monaural directivity, whereas for 1000 Hz, the advantage is substantial. This can be explained by the particular optimization underlying the binaural directional setting, which attempts to keep portions of the local signal necessary to maintain spatial orientation at an optimum level.
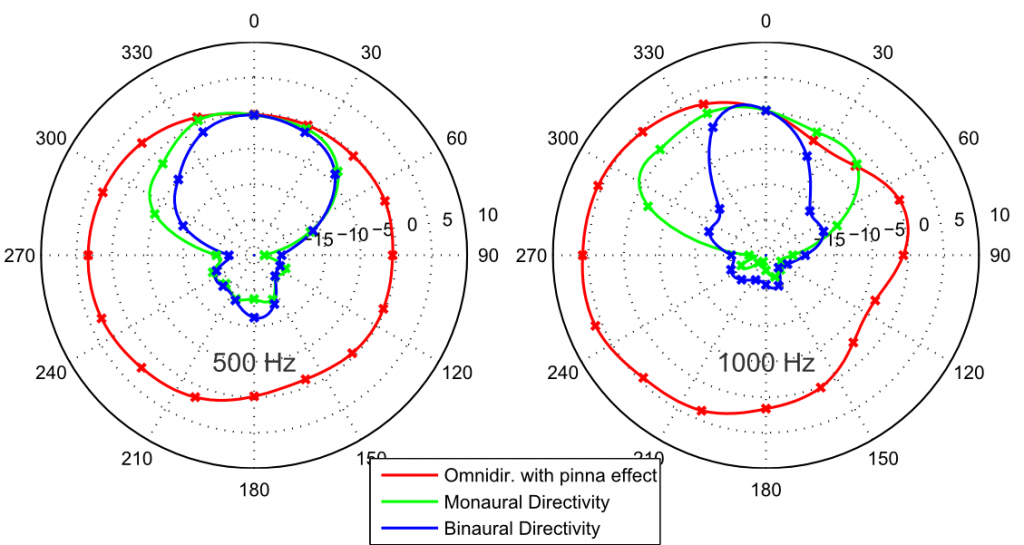
Figure 2. Polar patterns as measured with mini-BTE RIC (Pure carat 7bx) devices for f=500Hz (left) and f=1000Hz (right). The ITR is represented as the radial component in decibels, the angular components are the direction of arrival in degrees, where 0° is the frontal direction and 90° is the right side.
The difference in directivity across frequencies also can be observed in the sDI plot, which underlines the utility of the sDI metric. Figure 3 clearly shows the advantage of the binaural system in the higher frequencies.
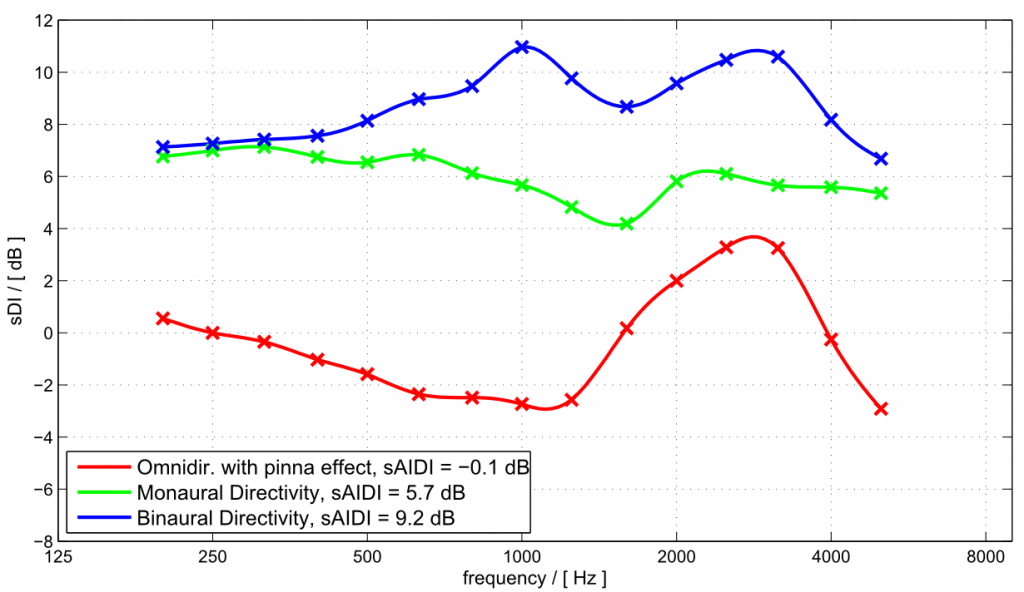
Figure 3. Sequential directivity index (sDI) across frequencies for mini-BTE RIC devices. Also shown in the figure legend is the sAI-DI calculation for each microphone mode.
As reviewed by Ricketts and Mueller10, and suggested by Killion et al11, the DI can be modified by adding speech weighting in an attempt to make it a better predictor of real-world performance. The original use of the AI-DI was based on using the importance functions taken from the Mueller-Killion Count-The-Dots audiogram12. Simply stated, the articulation-index-weighted directivity index (AI-DI) uses band importance weightings to assign more weight to the directional advantages for the frequencies most important for speech recognition (e.g., the DI for 2000 Hz is given a higher weighting that the DI for 500 Hz). We have, therefore, weighted the measured sDIs, in a similar manner1,7, in order to obtain the sAI-DI. The AI-DI (and now the sAI-DI) is expressed as a single average value. These values are included in the legend of Figure 3.
Comparison of Different Housing Types
There is a wide range of hearing aid housing types within one processing platform. The microphone inlet port positions and distances vary over the range of housings, which is why it is important to evaluate the respective directional performance of these different products. To provide a good reference, the respective measurements for an unaided KEMAR were also included. As depicted in Figure 4, the difference between housing types in the polar pattern is small, compared to the difference between the microphone modes. This is also reflected in the sDI metrics in Figure 5. Monaural directivity already provides a good directional benefit over a wide frequency range. One can also observe the natural directivity of the pinna in KEMAR’s sDI metrics. The directivity further increases in the binaural directional microphone mode, which results in another increase in sDI over most of the frequency range. The small variances between the measurements for different housing types is also reflected in a small variance in sAI-DI – see Table 1 for a comparison of the different products.
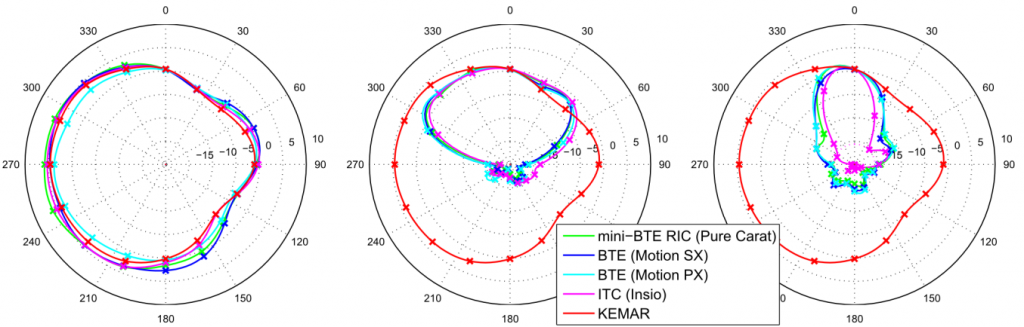
Figure 4. Measurements obtained with the hearing aids fitted to the left ear of the KEMAR. Interferer-to-Target ratio (ITR) for different housing types, measured at 1 kHz, compared to the KEMAR open ear. Left: Omnidirectional with pinna effect, Middle: Monaural directivity, Right: Binaural directivity.
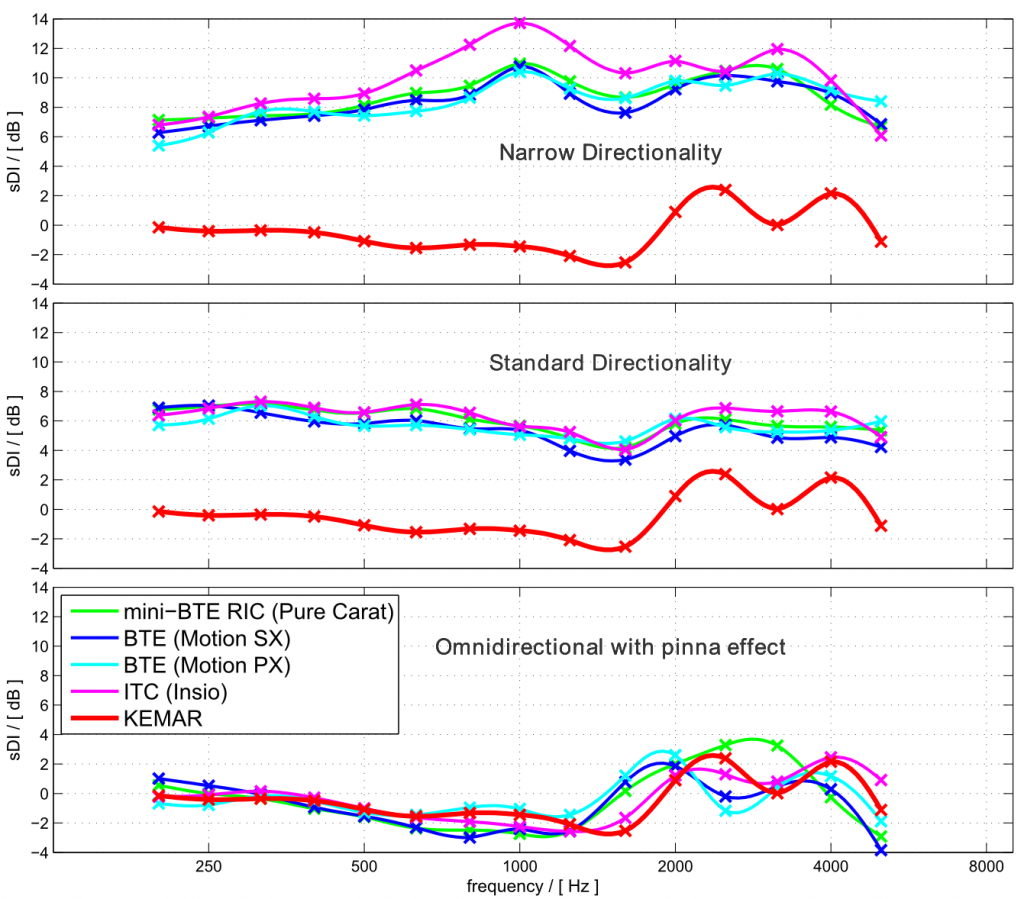
Figure 5. Sequential directivity index of various housing types and microphone modes.
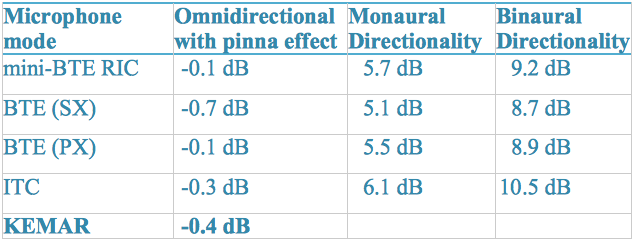
Table 1. sAI-DI Calculations for Different Housings and Microphone Modes and the KEMAR Open Ear.
Completely-in-the-Canal Devices with One Microphone
The binax Insio one-microphone device, a CIC product, also provides adaptive directionality. The axis of microphones in this case, is left-right, rather than the front-rear axis used for traditional directional products. This processing provides significant attenuation to signals from the sides and much of the rear hemisphere, which is achieved utilizing a bidirectional audio transmission between the hearing aids. As shown in Figure 6, starting from around 250 Hz, we see an increase in directional performance compared to omnidirectional, which becomes substantial throughout the frequency range. This is also reflected by the benefit in sAI-DI over omnidirectional (5.6 dB), which is relatively the same order of magnitude as traditional monaural directional microphone systems.
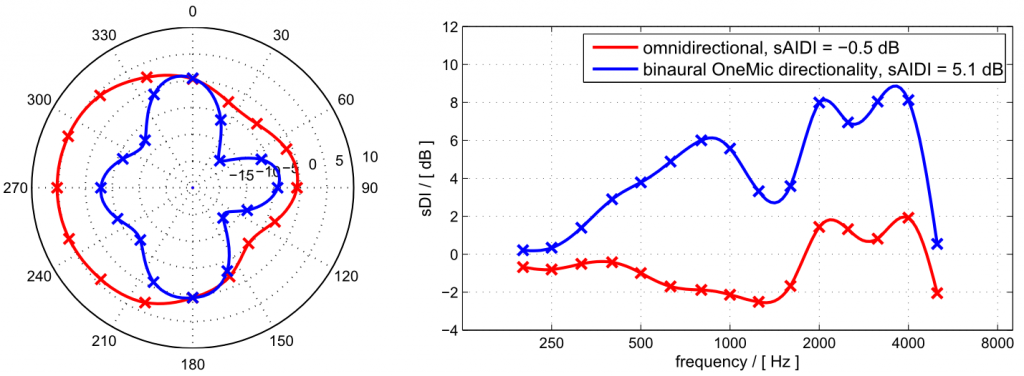
Figure 6. Polar pattern (ITR) for one-microphone binaural directionality at f=1kHz fitted to the left ear of the KEMAR (left panel). The sequential directivity index (sDI) is shown in the right panel.
Conclusion
We find an advantage in sAI-DI using the proposed assessment method for all housing types on the order of 5-6 dB for monaural and 9-10 dB for binaural microphone technologies for devices with two microphones. The deviation between housing types is small, which underlines the robustness of the proposed method.
For one-microphone CIC devices with binaural processing, we find an improvement in sAI-DI over the omnidirectional condition that is the same order of magnitude as the difference between omnidirectional and monaural directionality in two-microphone devices. In general, we believe the sDI approach to be a promising laboratory measure to evaluate and compare different directional technologies.
References
- American National Standards Institute. ANSI S3.35-2004: Method of measurement of performance characteristics of hearing aids under simulated real-ear working conditions. 2004.
- Elko GW and Pong ATN. A simple adaptive first-order differential microphone." IEEE Workshop on App Sig Proc to Acoustics; 1995.
- Kamkar-Parsi H, Fischer E, Aubreville A. Improving speech intelligibility in noise with binaural beamforming. Hear Rev 2014;21(10):42–45.
- Powers TA and Fröhlich M. Clinical results with a new wireless binaural directional hearing system. Hear Rev 2014;21(11):32–34.
- Hagerman B and Olofsson Å. A method to measure the effect of noise reduction algorithms using simultaneous speech and noise. Acta Acoust United Ac 2004;90(2):356–61.
- Wu YH and Bentler RA. Using a signal cancellation technique to assess adaptive directivity of hearing aids, J Acoust Soc Am 2007:122(1),496–511.
- Aubreville M and Petrausch S. Directionality assessment of adaptive binaural beamforming with noise suppression in hearing aids. Proc. of ICASSP 2015 [accepted].
- Holube I, Fredelake S, Vlaming M, Kollmeier B. Development and analysis of an international speech test signal (ISTS). Int J Audiol 2010;49(12):891–903.
- Ricketts TA and Henry P. Evaluation of an adaptive directional-microphone hearing aid. Int J Audiol 2002;41:100–12.
- Ricketts T and Mueller HG. Making sense of directional microphones. Am J Audiol 1999;8:117–26.
- Kilion M, Schulien R, Christensen L, et al. Real world performance of an ITE directional microphone. Hearing J 1998;51(4):24–38.
- Mueller HG and Killion M. An easy method for calculating the articulation index. Hearing J 1999;43(9):14–17.