How Asymmetric Directional Hearing Aid Fittings Affect Speech Recognition
The speech intelligibility benefits of directional microphones in hearing aids have been well documented.1 There is little disagreement that hearing aid directionality can be beneficial for hearing aid users in noisy situations in which a user’s goal is to understand speech. Hearing aid manufacturers have various strategies for optimizing microphone directionality including adaptive directionality, binaural beamforming, and automatic steering algorithms. Manufacturers have historically focused their development efforts on technical improvements to directional processing. Technical advances such as binaural beamforming make it possible to attenuate many decibels of background noise, but only under very specific situations. A common goal of most of these strategies is to reduce the need for the hearing aid wearer to change programs manually. By automatically adapting, hearing aids can help maintain speech intelligibility as the acoustic environment changes.
However, there are factors other than speech intelligibility to consider when choosing a directional algorithm, such as the algorithm’s influence on environmental awareness and localization abilities. Recently, Brimijoin et al., have shown that test subjects with hearing impairment were slower at orienting toward a source positioned far off-axis when listening with directional microphones compared to omnidirectional microphones.2 One strategy attempting to balance environmental awareness with the benefits of directional microphones is to use asymmetric directionality, where one of a user’s hearing aids is in an omnidirectional mode and the contralateral device is in directional mode. Bentler et al., and Cord et al., showed that speech intelligibility is similar for using asymmetric directionality compared to bilateral directionality,3,4 where both hearing aids are in directional mode. This may be explained by the brain utilizing the ear with a better signal-to-noise ratio for speech intelligibility. However, the absence of finding a significant performance difference between bilateral and asymmetric directionality in these 2 studies may be dependent on test setups and do not necessarily indicate that there are no performance differences between the directional approaches in other environmental conditions.
Hornsby and Ricketts pointed out 3 environmental conditions in which speech intelligibility was not optimal for asymmetric directionality.5 The authors tested 16 individuals with hearing impaired using bilateral omnidirectional microphones (“OO”), bilateral directionality (“DD”), and asymmetric directionality (“DO” or “OD”). Two variations of asymmetric directionality were tested, including the case in which the left hearing instrument was in directional mode and the right instrument in omnidirectional mode (“OD”) and the case in which the left instrument was in directional mode and the right instrument in omnidirectional mode (“DO”). Test subjects completed speech intelligibility tests in 3 different acoustic conditions: (1) noise sources surrounding the listener with a target talker in front, (2) noise sources to the left of the listener with a target talker in front, and (3) noise sources to the left of the listener with the target talker on the right.
Their results showed that in acoustic condition 1, bilateral directionality resulted in better speech intelligibility than either of the asymmetric directionality hearing aid modes. In acoustic condition 2, the authors found that performance was significantly worse, compared to bilateral directional, when the left ear was in omnidirectional mode and the right ear was in directional mode (“OD”), but not when the reversed asymmetric directional mode (“DO”). The hypothesized explanation for these results was that placing the left hearing aid, closest to the noise, in directional mode would attenuate the noise on the left, while not attenuating the speech. Results for acoustic condition 3 showed that the DO mode, but not the OD mode, resulted in significantly better speech intelligibility than the bilateral directional mode. This suggests that it was important for the hearing aid on the side of the speech to be in omnidirectional mode, in order to not attenuate the speech. Furthermore, there was no statistically significant difference between the results for OD than those of the DO mode. The explanation for the lack of differences was unclear, but they could be due to a lack of statistical power. The authors also used adaptive directional processing for the hearing aids that were in directional mode, so it was unclear exactly what the hearing aids were doing. For example, it was possible for the hearing aids to shift to an optimal directional pattern for a particular noise condition.
The present study was designed to gather additional data for noise conditions in which asymmetric directionality was hypothesized to result in non-optimal speech intelligibility. The noise conditions tested in the experiment were modified slightly compared to those of Hornsby and Ricketts in order to emphasize any potential differences between the results.5 The hearing aids used are based on a binaural directional strategy that utilizes both directional and omnidirectional microphone modes, in both symmetric and asymmetric modes. They are designed to provide automatic switching between these modes in order to optimize speech intelligibility, while at the same time providing the localization and sound quality advantages of omnidirectional microphones in conditions where speech intelligibility would not be compromised. This goal of this study is therefore to investigate the microphone modes in order to understand speech intelligibility differences between them.
Methods and Procedures
Participants
Twenty adults with normal-hearing and 19 adult test subjects with hearing impairment participated in the experiment. Test subjects in the normal-hearing group had air-conduction hearing thresholds of 20 dB HL or better in the frequency range 125 to 8000 Hz, with the exception of 3 subjects with 6 kHz thresholds of 25, 30, and 30 dB HL. Subjects with hearing impairment had mild-to-severe sloping hearing losses. In all but 2 of the cases, left and right air-conduction hearing thresholds were symmetric within 20 dB. Mean audiograms for the hearing-impaired subjects are shown in Figure 1. Additional details about the hearing-impaired test subjects are shown in Table 1.
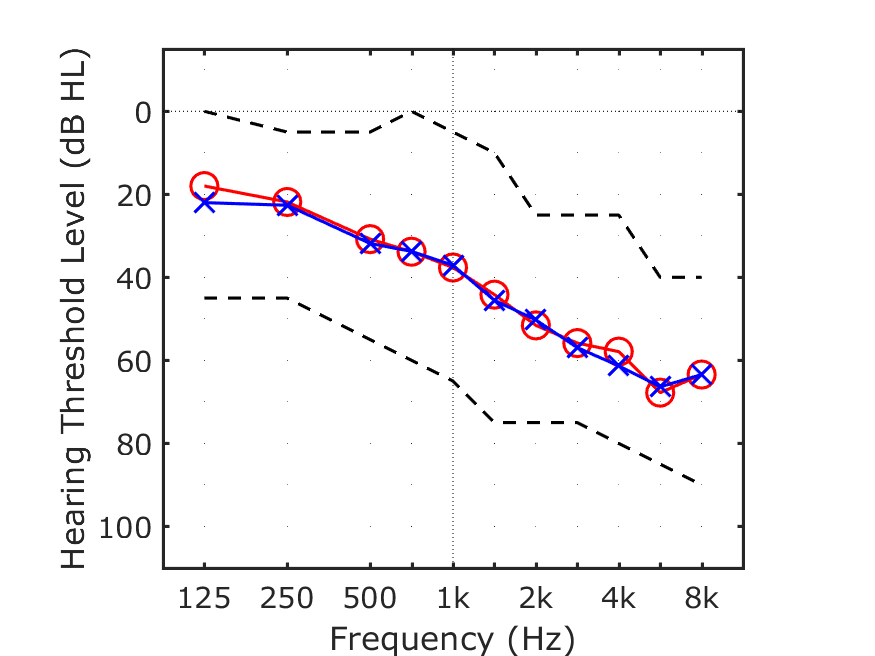
Figure 1. Mean left and right audiograms of hearing-impaired test subjects including minimum and maximum ranges for all ears.
Speech and Noise Configurations
Speech-in-noise testing was conducted using Dantale II, the Danish implementation of the Hagerman speech intelligibility test.6 The test adaptively varies the level of a speech signal, in the presence of speech-shaped background noise, in order to find the point at which 50% of the words are perceived correctly. The sentences are all 5 words long and are all in the form of a person’s name + an action verb + a numeral + an adjective + a direct object.
Four Tannoy i5 AW loudspeakers were arranged around the perimeter of a 2 m diameter circle. Speech material was presented through one of the loudspeakers, and speech-shaped noise was played from the 3 other loudspeakers. Two conditions were tested, as shown in Figure 2, where speech is presented from 0° and noise from 135, 180, and 225°. The listener faced 0° in Noise Condition A (NC A) and faced 90° in Noise Condition B (NC B). When measured at the position where the listeners would later sit, the levels of the speech-shaped noise was identical for each of the loudspeakers.
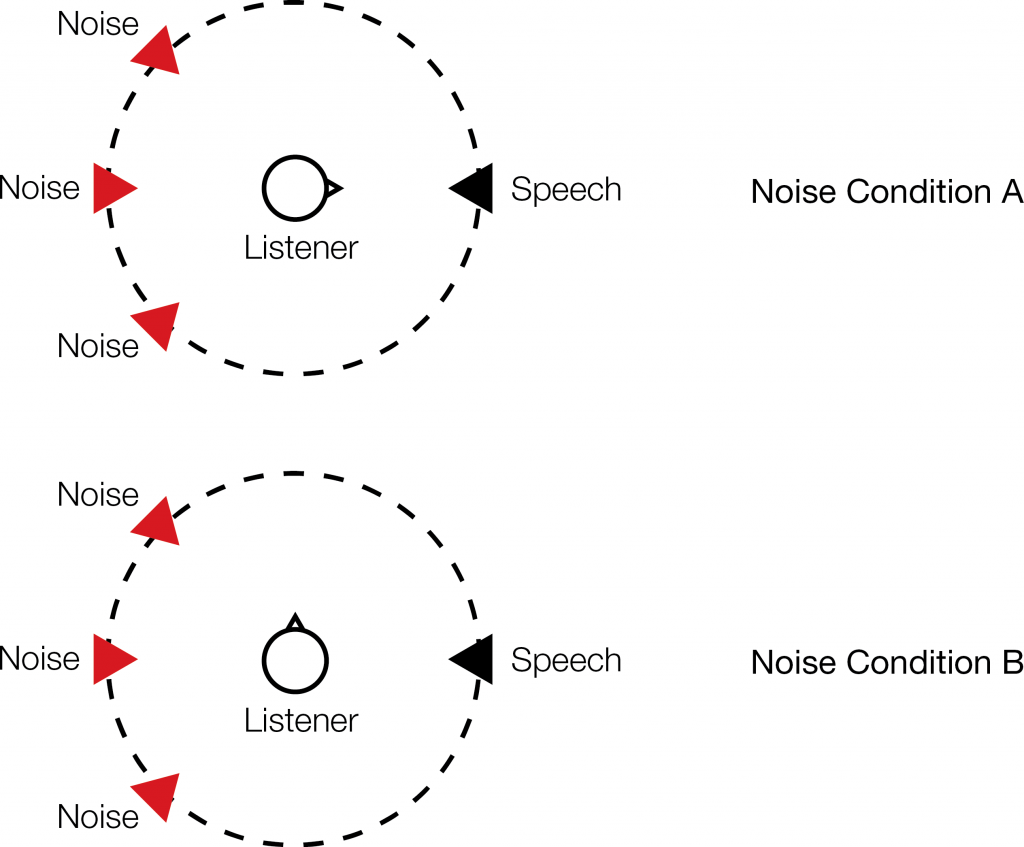
Figure 2. Diagram of the speech and noise configurations used in the experiments.
Amplification
All test subjects were fit bilaterally with ReSound Verso behind-the-ear hearing instruments, model VO-977. In order to maximize directional benefit, the instruments were fit using completely closed Comply-brand foam earmolds. The hearing-impaired test subjects were fit with the default ReSound Audiogram+ fitting rationale for inexperienced or experienced users, depending on their experience with amplification. Fine-tuning was limited to addressing subjective complaints of loudness. Normal-hearing test subjects were fit with a 10 dB flat insertion gain, as estimated by the fitting software. For all test subjects, adaptive hearing aid algorithms were turned off to the widest extent possible. These included expansion, noise reduction, wind noise reduction, and environmental gain offsets. The only adaptive algorithms left on were those relating to feedback control for the hearing-impaired test subjects.
In order to help ensure the hearing aids were in the desired directionality modes, the automatic environmental steering functionality was disabled. All hearing aids were fit with 4 programs: Omni/Omni, Omni/Directional, Directional/Omni, and Directional/Directional, for the Left/Right hearing aids respectively. Hereafter, these directionality configurations will be referred to as OO, OD, DO, and DD. The directional modes were set to be directional across as much of the frequency range as possible (The ReSound Aventa fitting software setting called “Directional Mix”, which allows the hearing aid to use omnidirectional processing in the low frequencies and directional processing in the high frequencies, was set to ”High.” This made directionality active in the largest possible range of frequencies.), making the hearing aids effectively directional above 500 Hz.
For Noise Condition A, test subjects completed speech intelligibility tests for OO, OD, and DD modes. Because the test setup was symmetrical, only one of these asymmetric directional settings was tested – the OD configuration. For Noise Condition B, the OO, OD, DO modes were tested. Prior to each round of testing, test subjects also completed 2 unaided practice rounds. This was done in order to reduce the impact of inexperience with the speech intelligibility test.7
Real-ear measurements were performed for all test subjects in order to ensure that the earmolds were tightly sealed and that the level of the amplified sound from the hearing aid was dominant over any direct sound reaching the ear canal. Adjustments to earmold size and placement were made when leakages were detected.
Results
Speech reception thresholds for normal-hearing and hearing-impaired subjects are shown in Figure 3 and Figure 4, for Noise Condition A and Noise Condition B respectively. The patterns of performance were similar across the 2 groups. For Noise Condition A, the poorest thresholds were seen for the OO program and the best with the DD. The asymmetric program resulted in performance in between that of OO and DD. However, in Noise Condition B, optimal performance was seen for the OO program, with the directional programs both performing worse. The OD program resulted in the worst performance.
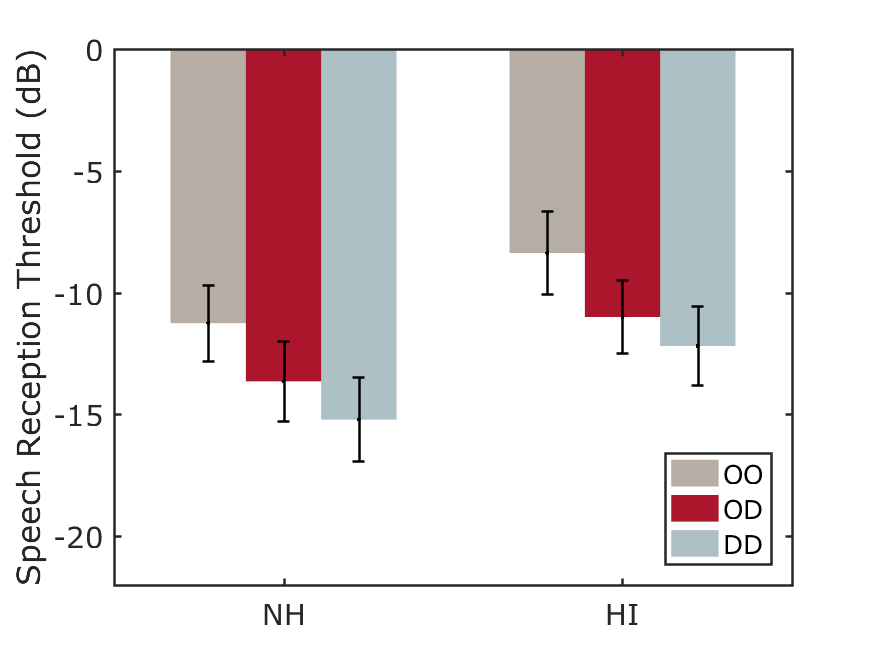
Figure 3. Speech reception thresholds for Noise Condition A. Results are shown for normal hearing (NH) and hearing impaired (HI) test subjects, for each of the hearing aid directional modes.
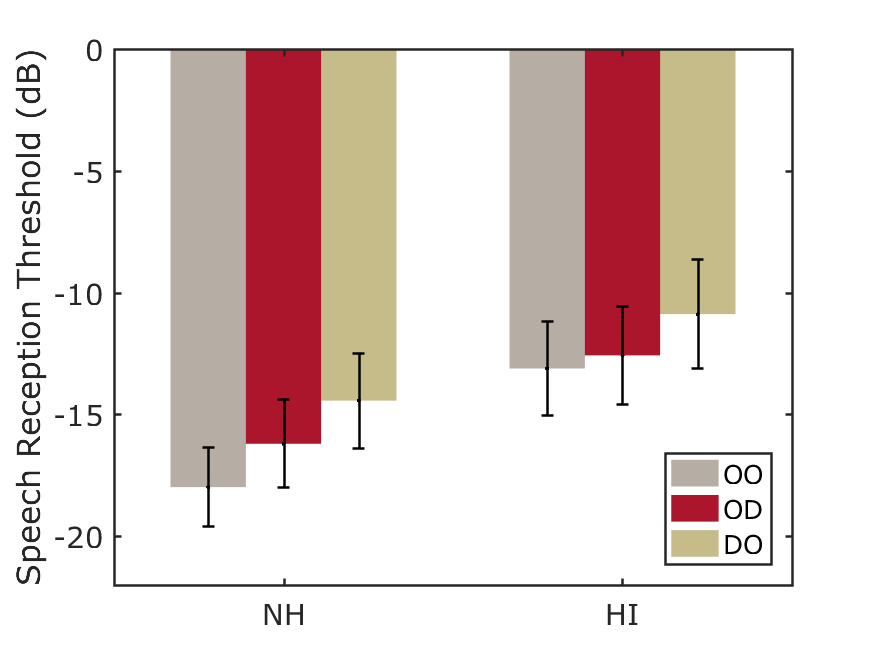
Figure 4. Speech reception thresholds for Noise Condition B. Results are shown for normal hearing (NH) and hearing impaired (HI) test subjects, for each of the hearing aid directional modes.
For the statistical analysis, microphone mode was considered a nested variable inside noise condition due to the fact that different combinations of directionalities were tested in the 2 noise conditions. A three-factor analysis of variance (ANOVA) revealed a main effect of test subject group (F1,37= 51.15, p < 0.001), noise condition (F3,185 = 194.20, p < 0.001), microphone mode as a function of noise condition (F2,152.87 = 30.62, p < 0.001), as well as a significant interaction between test subject group and noise condition (F3,185 = 7.39, p < 0.001). The interaction between test subject group and microphone mode was not significant (F2,185 = 3.09, p = 0.43).
The results for each noise condition were then analyzed separately. In Noise Condition A, a two-factor ANOVA showed a main effect of test subject group (F1,37 = 37.59, p < 0.001) and microphone mode (F2,74 = 186.12, p < 0.001). There was no significant interaction between test subject group and microphone mode (F2,74 = 0.44, p = 0.65). In Noise Condition B, a two-factor ANOVA showed a significant main effect of test subject group (F1,37 = 52.67, p < 0.001), microphone mode (F2,74 = 74.68, p < 0.001), and the interaction between test subject group and microphone mode (F2,74 = 4.78, p < 0.05).
Further ANOVAs were conducted, separating the 2 subject groups, for each noise condition. For the normal-hearing subjects in Noise Condition A, a significant effect of microphone mode was seen (F2,38 = 266.29, p < 0.001). Similarly, for the hearing-impaired subjects in Noise Condition A, a significant effect of microphone mode was seen (F2,36 = 72.43, p < 0.001). For Noise Condition B, a significant effect of microphone mode was also seen for the normal-hearing test subjects (F2,38 = 63.01, p < 0.001) and the hearing-impaired test subjects (F2,36 = 25.78, p < 0.001).
In order to investigate which microphone modes were significantly different, a series of planned comparisons, using single-factor repeated-measures ANOVAs, were then performed for each noise condition and each subject group. The results are shown in Table 2. All comparisons were statistically significant, with each directionality mode being significantly different than OO and with each directionality mode being different than one another (OD vs DD for Noise Condition A and OD vs DO for Noise Condition B).
Figure 5 and Figure 6 show the benefit of each of the directional modes over the OO configuration, for Noise Condition A and B respectively. In Noise Condition A, for both normal-hearing and hearing-impaired subjects, both the asymmetric directionality and bilateral directional patterns resulted in better performance than bilateral omnidirectional. The bilateral directional setting provided the most benefit. In Noise Condition B, the DO asymmetric setting performed better than OD for both subject groups. However, the bilateral omnidirectional pattern resulted in better performance than either of the asymmetric patterns. This was expected for the OD asymmetric pattern, but the explanation for the comparison of the DO to OO patterns is less straightforward. However, the difference between OO and OD is less than 1 dB for the hearing-impaired test subjects and thus unlikely to be clinically significant.
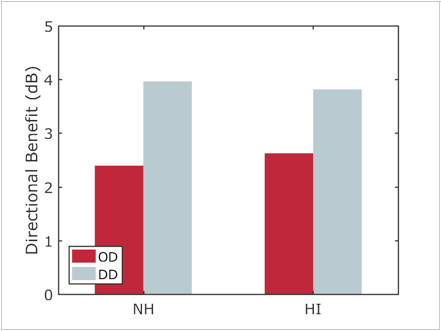
Figure 5. Directional benefit of using OD and DD directional programs relative to OO in Noise Condition A. Benefit is shown for normal hearing (NH) and hearing impaired (HI) test subjects. All program configurations were significantly different from one another.
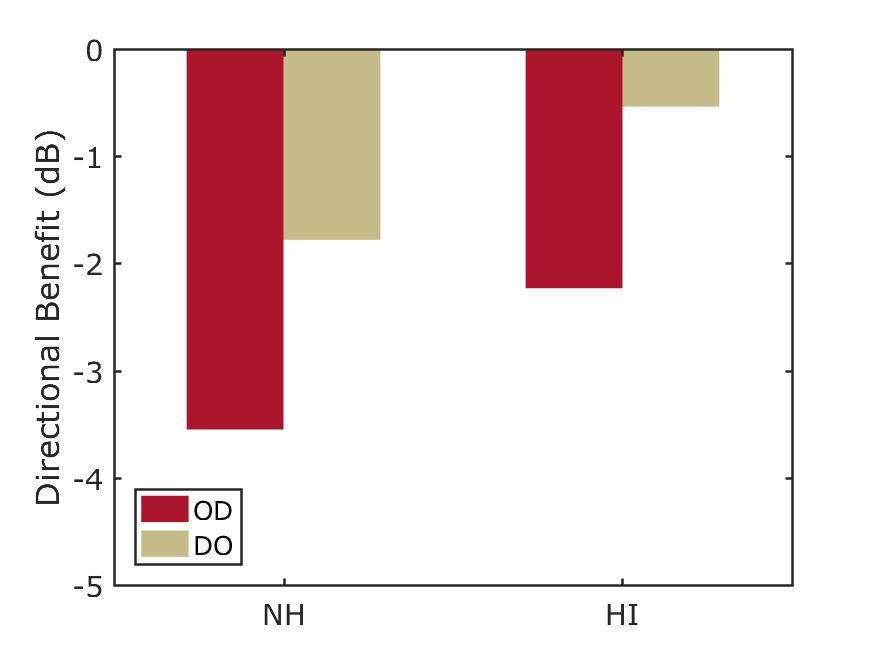
Figure 6. Directional benefit of using OD and DO asymmetric directional programs compared to OO in Noise Condition B. Benefit is shown for normal hearing (NH) and hearing impaired (HI) test subjects. All program configurations were significantly different from one another.
Discussion
These results confirm those found by Hornsby and Ricketts for Noise Condition A, for which they tested in a similar setup.5 The results provide even greater statistical significance, possibly due to the differences between the setups, speech intelligibility tests, and number of test subjects. The results can furthermore be generalized to normal-hearing listeners wearing hearing aids.
Hornsby and Ricketts also tested a setup similar to Noise Condition B, but with the speech loudspeaker located closer to the listener.5 The authors did not find a difference in performance between the bilateral omnidirectional mode and the asymmetric DO mode, but a statistically significant difference was found in the present experiments for both the normal-hearing and hearing-impaired test subjects. Furthermore, Hornsby and Ricketts did not find a difference between the OD and DO asymmetric modes, but the present experiments found a small significant difference, again for both the normal-hearing and hearing-impaired test subjects. The explanation for the differences in these findings could lie in the greater statistical power offered in the present experiments by having used a greater number of test participants. However, there may be other explanations due to differences in the physical setups. Therefore, the reader should use caution when comparing the 2. Rather, the results may be seen as complementary. The position of speech loudspeaker in closer proximity to the listener’s head, in the setup of Hornsby and Ricketts, may have served to increase the amount of attenuation provided by the physical size of the head, and thereby better isolate the left and right ears to the loudspeakers on their respective sides.5 In the present experiments, the ears were possibly less effectively separated from the loudspeakers on their respective sides, perhaps leading to an influence on the left ear in detecting speech from the right side of the head. When the hearing aid on the left ear was set to directional mode, the influence of the attenuation to the speech on the right-hand side may have caused the degradation in speech reception thresholds compared to when the left-hand hearing instruments was set to omnidirectional mode.
Speech intelligibility and environmental awareness are factors that are largely determined by the availability of appropriate auditory input to the ears. Ensuring that the brain receives appropriate input is therefore a critical aspect in providing for natural auditory perception. This could be taking advantage of an improved signal-to-noise ratio for sound coming from in front, but it could just as well be audibility for sound coming from other locations. Traditional directional solutions of bilateral hearing instruments can optimize audibility and beamforming characteristics, but they do not necessary lead to a natural, binaural processing of sound. The underlying assumptions made by such systems are that the signal of interest is stable and predictable, which is not the case in the majority of real-world listening situations. The brain’s remarkable ability to shift attention, based on the hearing aid wearer’s intent, is only usable if the necessary binaural input is provided. This study shows which ReSound microphone modes benefit speech intelligibility, while also demonstrating the importance of the binaural microphone modes that allow the user to decide on the signal of interest.
Conclusions
The experiments here have examined 2 acoustic situations that were previously shown by Hornsby and Ricketts to be challenging for fixed, asymmetric directional programs.5
- The results confirm the importance of bilaterally coordinated adaptive switching between asymmetric and symmetric directional configurations.
- The results additionally serve as a reminder of the importance of the standard, bilateral omnidirectional program. With a talker on one side of a listener, it appears that a bilateral hearing aid fitting should be adaptively switched to a symmetric omnidirectional program for optimal speech intelligibility.
- Furthermore, the results confirm that normal-hearing and hearing-impaired subjects show similar trends in these noise conditions. Although the results are not identical in magnitude, the similarity suggests that evaluation of directionality settings on normal-hearing test subjects may be reasonable for the purposes of finding potential differences between directional settings.
Acknowledgements
The authors would like to think Derya Schjerlund Ceylan, Astrid Haastrup, Lotte Hernvig, and Peder Thyme for collecting the experimental data.
References
- Mueller HG and Ricketts TA. Directional-microphone hearing aids: An update. Hear J 2000;53:10–19.
- Brimijoin WO, Whitmer WM, McShefferty D, et al. The effect of hearing aid microphone mode on performance in an auditory orienting task. Ear Hear 2014;35:204–12.
- Bentler RA, Egge JL, Tubbs JL, et al. Quantification of directional benefit across different polar response patterns. J Am Acad Audiol 2004;15:649–59.
- Cord M, Surr R, Walden B, Dittberner A. Asymmetric directional microphone fittings. Paper presented at the American Academy of Audiology 17th Annual Convention, Washington, D.C.; 2005.
- Hornsby BW and Ricketts TA. Effects of noise source configuration on directional benefit using symmetric and asymmetric directional hearing aid fittings. Ear Hear 2007;28:177–86.
- Wagener K, Josvassen JL, Ardenkjær R. Design, optimization, and evaluation of a Danish sentence test in noise. Int J Audiol 2003; 42:10–17.
- Hernvig LH and Olsen SO. Learning effect when using the Danish Hagerman sentences (Dantale II) to determine speech reception threshold. Ear Hear 2005;44:509–12.