The Vestibulo-Ocular Reflex
I want you to try something: sit at approximately arm’s length from this article and shake your head back and forth (like saying “no”) while staring at THIS word.
Go ahead, try it!
Now, give yourself the thumbs-up with your right hand. Hold that thumb at arm’s length and stare at your thumbnail. Now bob your head up and down (like saying “yes”).
Could you read that word clearly and see your thumbnail clearly? Even when your head was moving? Then congratulations – you have a functioning vestibulo-ocular reflex (VOR)! This important reflex generates rapid compensatory eye movements of equal velocity, but opposite direction to, head movements and thus allows us to move around and see clearly at the same time, through fast foveal image stabilisation. Without the VOR, life would look as though we were watching it through a hand-held video camera.
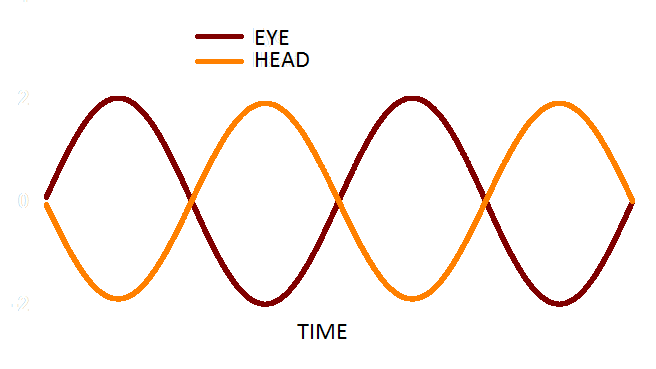
Figure 1. In a functioning VOR, head (orange) and eye (red) movement are equal in amplitude and opposite in direction.
The easiest way to understand how our VOR works is through a diagram. Figure 2 illustrates the VOR pathway for the lateral semicircular canal. The sequence begins with a head turn to the right (top of diagram). During this rightward head rotation, movement of endolymph on the right side induces a deflection of the cupula whereby the stereocilia bend towards the kinocilium. The movement of stereocilia is away from the kinocilium in the opposing ear. As a result of this “push-pull principle,” the activity of the right primary afferent neurons increases and the activity of left primary neurons decreases, simultaneously. In other words, head rotation to the right results in depolarization of the right horizontal canal and hyperpolarization of the left horizontal canal. This information then travels to the vestibular nuclei where there is excitation in the right and inhibition in the left. Following this, the information travels to the level of the extraocular motor nuclei. This pattern of extraocular motor activity results in eye muscle contraction: contraction of the right medial rectus and the left lateral rectus, as well as relaxation of the left medial rectus and the right lateral rectus muscles; thus generating an eye movement to the LEFT.
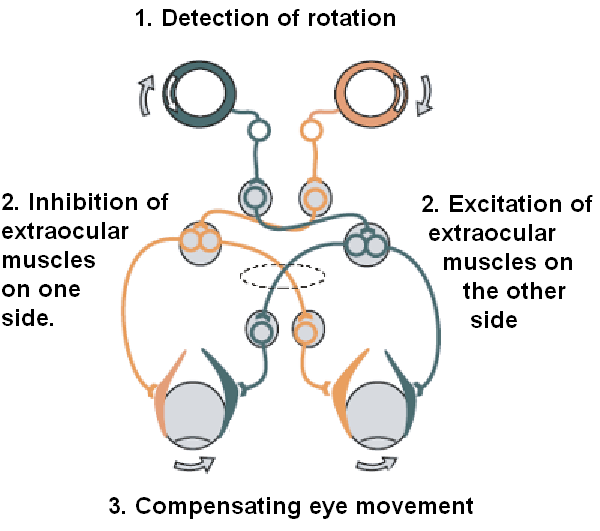
Figure 2. Simple schema of the VOR pathway.
The above description outlines the pathway relating to the lateral semicircular canal, only; however, the VOR works in the superior and posterior semicircular canals as well. Furthermore, the VOR has contributions from the otolith organs (utricle and saccule). This is demonstrated by the fact that there are both rotational and translational aspects of the VOR. When there is rotational (angular) head movement, a visual fixation point is stabilized by rotating the eyes about the same axis, but in the opposite direction, primarily using input from the semicircular canals (RVOR). With translational (lateral) movement, a visual fixation point is maintained by rotating gaze direction in the opposite direction by an amount that depends on distance, using information primarily derived from the otoliths (TVOR).
Why Do We Need Our VOR?
Patients with reduced VOR function cannot generate sufficient compensatory eye movement, meaning their eye movements are not large enough to compensate for their head movements. A common complaint from these patients is the experience of visual blurring while in motion (e.g., walking, driving). This is called oscillopsia. There is an infinite list of examples demonstrating the necessity of our VOR. Head and body movements are constantly occurring, and a focused foveal image during those movements will prevent a blurred image being presented to the brain.
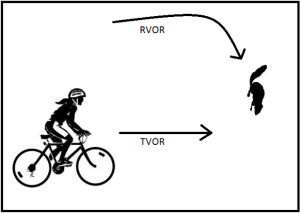
Figure 3. A marauding squirrel.
Here’s an example of how you might be using your RVOR and TVOR. Imagine that you are on your bicycle on a beautiful summer day. All of a sudden a squirrel darts out from the left and appears to be running directly across your path. You need to keep the image of the squirrel focused on your fovea as you decelerate to avoid a collision. In order to complete this complicated manoeuvre successfully, your RVOR (turning your head to follow the squirrel’s progress) and TVOR (maintaining gaze on the squirrel while decelerating) must both be working. This is obviously very important for the health of the squirrel!
How Do We Test the VOR?
Head Impulse Testing (HIT)
The head impulse (or head thrust) test was first described by Halmagyi and Curthoys in 1988. The HIT is a commonly used manoeuvre, as it can be safely and quickly completed at bedside with the patient. It is used primarily for identifying VOR deficits of the lateral semicircular canals, but can also be used for the superior and posterior semicircular canals. It can reliably identify unilateral and bilateral peripheral lesions.
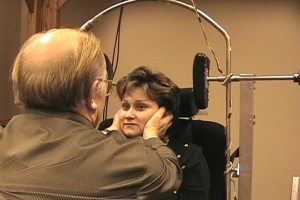
Figure 4. The head impulse test, as completed bedside. The patient maintains a steady gaze while a head thrust is performed.
In order to complete the test, the patient is asked to fixate on a target while the examiner rotates the head. The starting position should be such that the patient’s head is turned slightly past the midline (15–20°), and then should be thrust to the opposite side using abrupt movements. The movements should remain small (the patients head should not move very far either to the left or right), and the movement direction should be unpredictable. An abnormal result is indicated when the patient is unable to maintain a steady gaze on the target during a head thrust, and thus must complete a catch-up saccade. If the patient has a unilateral loss, the abnormal HIT result will occur with a thrust towards the side of lesion, and in a bilateral loss, the abnormal result will occur with head thrusts in both directions. A catch-up saccade may occur during the head movement (covert saccade) or after the head movement (overt saccade). Given that covert saccades cannot be seen with the naked eye and overt saccades may be difficult to identify (particularly for inexperienced testers) the bedside version of this test should be considered the least reliable method of measuring the VOR.
Two methodologies are available for capturing both types of saccades with a very high level of precision. These are the magnetic scleral search coil and the video head impulse test (vHIT) system.
The magnetic scleral search coil is comprised of a silicone annulus with a fine copper wire embedded along its outermost edge, which is placed on the eye similarly to a contact lens. The testing takes place within an electromagnetic field, which detects movements of the eye through voltage changes induced by movement of the coil within this field. The eye movement is referenced to the head movement using an additional coil affixed to a “bite-block” placed in the mouth. The scleral search coil is capable of accurately capturing very rapid (>300°/s) eye movements, which make it possible to observe both covert and overt saccades during HIT. In addition to noting any saccades, the data is analysed to produce gain values across velocities. Reduced gain would be another indicator of impaired vestibular function, either unilaterally or bilaterally. The scleral coil is still considered the “gold standard” for high velocity testing of the VOR; however, it is invasive and costly to administer. Scleral search coil testing can be completed in any of the three planes of the semicircular canals, and can also be used to measure the translational VOR through the use of controlled, small lateral head movements.
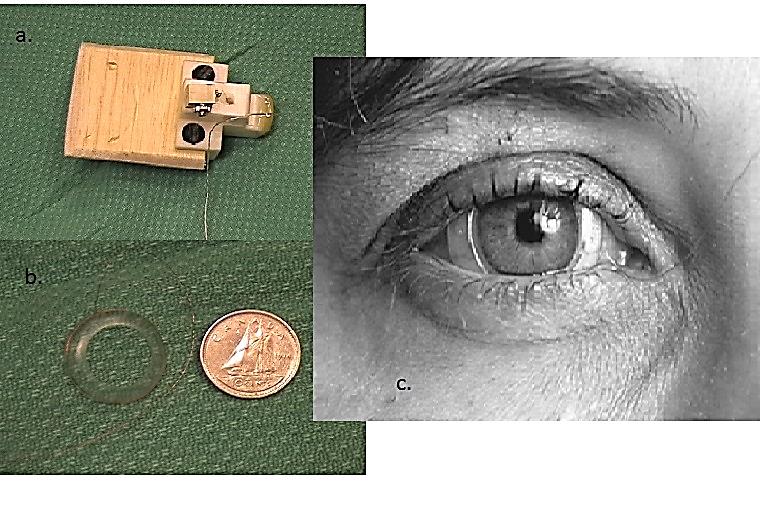
Figure 5. (a) the head coil (“bite block”); (b) scleral coil; (c) scleral coil on an eye.
The vestibular Head Impulse Test (vHIT) uses high-speed video to capture eye movements. For this test, the patient wears a pair of lightweight goggles fit snugly to the head, and affixed with a video camera and a built-in digital gyroscope for measuring head movements. Similarly to the scleral search coil, the vHIT produces gain values for all six semicircular canals as well as overt and covert saccades, for an accurate measure of impaired VOR function. The vHIT is capable of capturing moderately fast eye movements; however, impulses exceeding 200°/s have not yet been validated. Currently, vHIT testing is gaining popularity, as it is fast to perform and user-friendly, and is much more clinically practical than the scleral search coil.
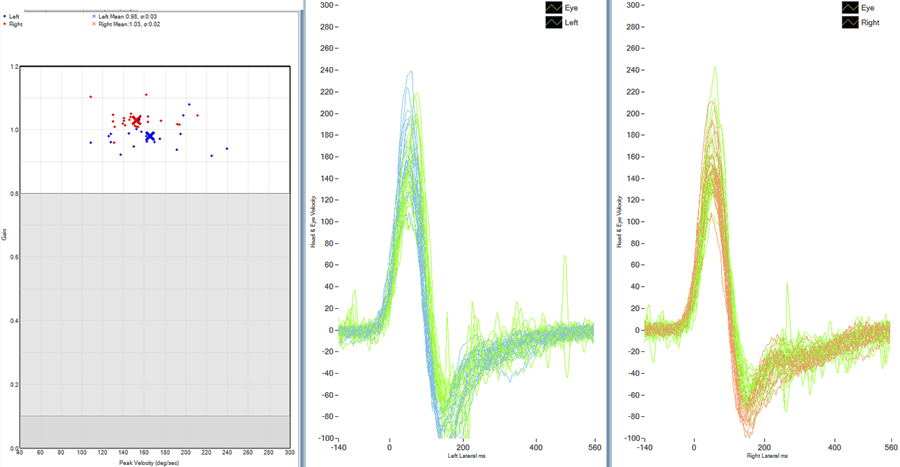
Figure 6. Normal vHIT. The graph on the left panel displays the gain values of velocities tested for each impulse (red = right, blue = left). Each “dot” represents an individual head impulse. The displays in the centre and on the right show the velocity across time for all of the head impulses (green = eye movement left/right, blue = head left, red = head right). It appears normal, as the head and the eye movements gains approach 1.0, and there are no apparent saccades.
Rotational Chair Testing
Another method of testing the VOR is the computerized rotational chair test. This test causes stimulation of both canals at once, which provides information about the function of the vestibular system as a whole during head rotation. There are several rotational chair tests that are used clinically to assess VOR function.
Sinusoidal harmonic acceleration testing
The chair is moved in a sinusoidal fashion, with successive increases in frequency, usually from 0.01 to 2 Hz. This allows for the analysis of eye movements in response to various angular accelerations. This test provides information about the VOR at lower velocities than the scleral coil and the vHIT, as the velocity of rotation does not typically exceed 40–60°/s. Abnormal responses can be identified through abnormalities of gain or phase (e.g., eye movements leading the head movements). As with the vHIT, we expect a gain of approximately 1.0 for a normally functioning VOR.
Constant angular acceleration
For this test, the chair must be able to rotate 360°. The rotational velocity of the chair is increased at a constant acceleration until a maximum velocity is reached. The chair rotates at a steady velocity for a time, after which deceleration occurs gradually. Nystagmus is measured during acceleration and deceleration. This test can be completed in both directions.
Impulse angular acceleration
For this test, the chair rotates 360° at a constant rate until the chair rotation is abruptly stopped, which causes a brief period of nystagmus. The slow-phase velocity of the nystagmus is measured. The test is completed several times in both directions, and the results are plotted across time. Both gain and “time-constant velocity” (the time for slow-phase velocity to drop by 37%) are measured.
Velocity step test
In this test, the chair is accelerated very rapidly (100–200°/s) and this velocity is maintained for 60 seconds, after which rapid deceleration occurs. This deceleration is perceived as rotation in the opposite direction. The gain and time constants values are measured. Results from this test can also help in the identification of unilateral peripheral loss.
Caloric Testing
In 1914, Robert Bárány received the 1914 Nobel Prize in Physiology or Medicine for his work on the physiology of the vestibular system. According to his work, caloric irrigation creates a temperature gradient across the lateral semicircular of the stimulated ear, causing changes in density of the endolymph. This creates nystagmus by causing deflections in the cupula, which changes the neural firing rate from the lateral labyrinth of the test ear. It is believed that caloric stimulation is analogous to a very slow head rotation, and therefore initiates the VOR. However, arguments against this theory have been put forward, such as the fact that a caloric response can be elicited from "dead" ears, the duration of the caloric response (to both cold and warm stimuli) is greater in the face-up than in the face-down position, and caloric nystagmus can occur in a zero-gravity environment. To date, a definitive mechanism does not yet exist to explain the nystagmus produced from caloric testing.
What To Do With the VOR Results?
VOR testing should be considered an important part of any vestibular test battery, as it contributes meaningful diagnostic information that cannot be obtained through other vestibular tests. If a patient is diagnosed with a VOR deficit of one or both vestibular end organs, vestibular rehabilitation may be recommended, depending on the patient’s diagnosis.
Bibliography
Halmagyi GM, Curthoys IS. A clinical sign of canal paresis. Arch Neurol 1988;45:737–39.
Jacobson GP, Shepard NT. Balance function assessment and management, San Diego: Plural Publishing; 2008.
Kaplan DM, Marais J, Ogawa T, et al. Does high-frequency pseudo-random rotational chair testing increase the diagnostic yield of the eng caloric test in detecting bilateral vestibular loss in the dizzy patient? Laryngoscope 2001;111(6):959–63.
Maes L, Dhooge I, De Vel E, et al. Normative data and test-retest reliability of the sinusoidal harmonic acceleration test, pseudorandom rotation test and velocity step test. J Vestib Res 2008;18(4):197–208.
Pothier D and Falls C. Vestibular function testing. (in press).
Schmid-Priscoveanu A, Böhmer A, Obzina H, and Straumann D. Caloric and search-coil head-impulse testing in patients after vestibular neuritis. J Assoc Res Otolaryngol 2001;2(1):72–78.
Stahle J. Controversies on the caloric response. From Bárány's theory to studies in microgravity. Acta Otolaryngol 1990;109(3-4):162–7.
Wuyts F. Principle of the head impulse (thrust) test or Halmagyi head thrust test (HHTT), B-ENT 2008;4(8):23–25.