Mysteries of the Hearing Brain
We know that patients can be quite overwhelmed by amplification when they first start wearing hearing aids, especially for sounds in the high-frequency region, where hearing loss is typically most severe. I used to tell my patients that it would be like coming out of a movie theatre in the middle of the day – the eyes need to adjust to the bright sunshine. Of course, that is not quite an accurate analogy as the pupils adjust dilation for light/darkness on a faster timescale than the impaired auditory system adjusts to increases in loudness. When counseling our patients, it may be helpful to understand the mechanisms involved in adjusting to increased loudness.
Sensorineural hearing loss (SNHL) usually results in limited tolerance for loud sounds. Recruitment is a well-known phenomenon that can account at least partially for the reduced dynamic range seen in individuals with SNHL. The outer hair cells serve as cochlear amplifiers. This amplification is regulated by abundant efferent connections that project from the brain to the cochlea.1,2 When there is a loss of outer hair cells, higher signal levels are required for detection of the signal. The increased signal level then activates a broader region of the cochlea, triggering cochlear amplification of the signal. A small increase of 10 or 20 dB in signal intensity may, therefore, result in comfort ratings that change from barely audible to loud.
The dynamic range may also be reduced by neural mechanisms that compensate for reduced auditory input. The brain likes to maintain a stable rate of neural firing, a process known as homeostasis. This stable firing rate is maintained by altering the balance of excitatory and inhibitory neurotransmission so that there is an overall increase in excitability. Increased excitability may explain why you might observe larger amplitudes when recording evoked potentials to suprathreshold auditory signals in an individual with SNHL compared to an individual with normal hearing.
The frequency-following response (FFR) is an evoked potential that is recorded from the scalp using as few as three electrodes. The FFR to speech has an onset of approximately 7 ms,3 reflecting a brainstem/midbrain origin, and its response to the stimulus vowel region may receive cortical contributions as well.4 Anderson et al.5 compared FFRs to the speech syllable /da/ in older adults with mild-to-moderate SNHL who were matched in age to older adults with normal hearing. They found that responses to the 100-Hz fundamental frequency (voice pitch) were larger in the group with SNHL than in the group with normal hearing (Figure 1). This enhancement may not be beneficial for perception, however, because the higher-amplitude low-frequency vowels may mask the lower-amplitude high-frequency consonants.
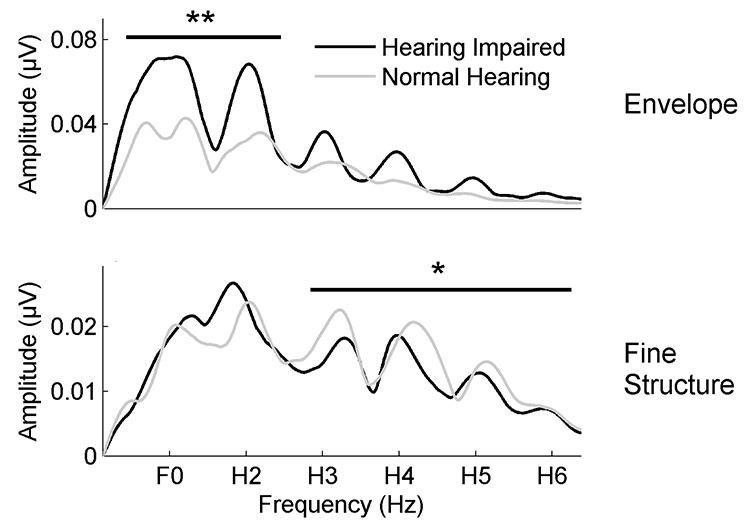
Figure 1. Top panel: Average responses to the speech envelope show larger responses to the fundamental frequency (F0) and second harmonic (H2) in older hearing-impaired participants (black) compared to older normal-hearing participants (gray). Bottom panel: Average responses to the speech fine structure show larger responses to the third through sixth harmonics (H3-H6) in older normal-hearing compared to older hearing-impaired participants. *p<0.05, **p<0.01. (Adapted with permission from Anderson et al. (2018) with permission from Frontiers)
Enhanced responses to the speech envelope are also observed in cortical responses, even in older adults with normal hearing. Presacco et al. recorded magnetoencephalographic (MEG) responses to one-minute sentences in young normal-hearing, older normal-hearing, and older hearing-impaired participants.6 Neural fidelity can be estimated by reconstructing the speech envelope from cortical activity and then correlating the reconstructed speech envelope to the envelope of the actual speech signal. Higher correlation values represent higher neural fidelity. Both groups of older adults had higher reconstruction r values than younger adults (Figure 2). In summary, these two studies show that exaggerated amplitudes at both cortical and brainstem levels may provide an explanation for the difficulty older adults experience when adjusting to amplified speech.
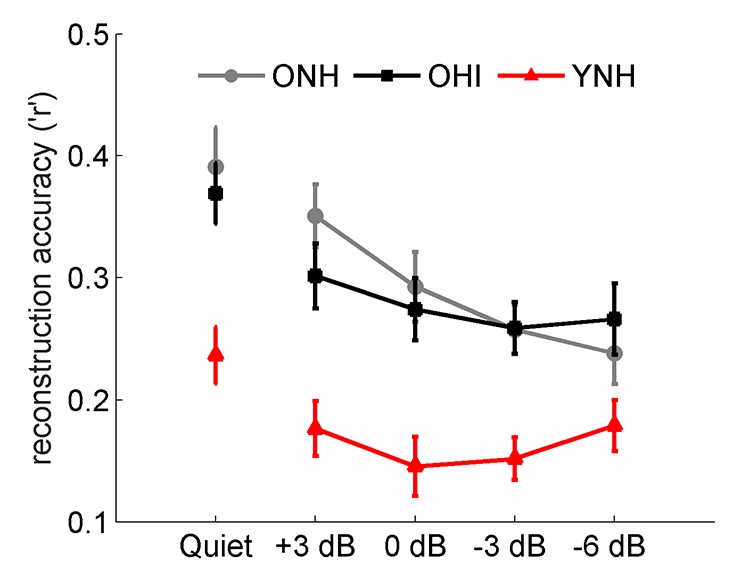
Figure 2. Older adults, both with normal hearing (ONH, black) and with hearing loss (OHI, gray), have exaggerated cortical responses compared to young adults with normal hearing (YNH, red) in both quiet and competing speech conditions. (Adapted with permission from Presacco et al.6 with permission from PloS ONE).
Fortunately, the older brain retains some degree of neuroplasticity. The re-introduction of sound through auditory training may change the weighting of speech cues by reducing response amplitudes to the fundamental frequency. Anderson et al.5 recorded FFRs to the speech syllable /da/ in older adults with normal hearing and hearing loss before and after forty hours of auditory-cognitive training. They found that training reduced the FFR fundamental frequency, and that pre-existing amplitude differences between ONH and OHI groups were significantly reduced following training (Figure 3).
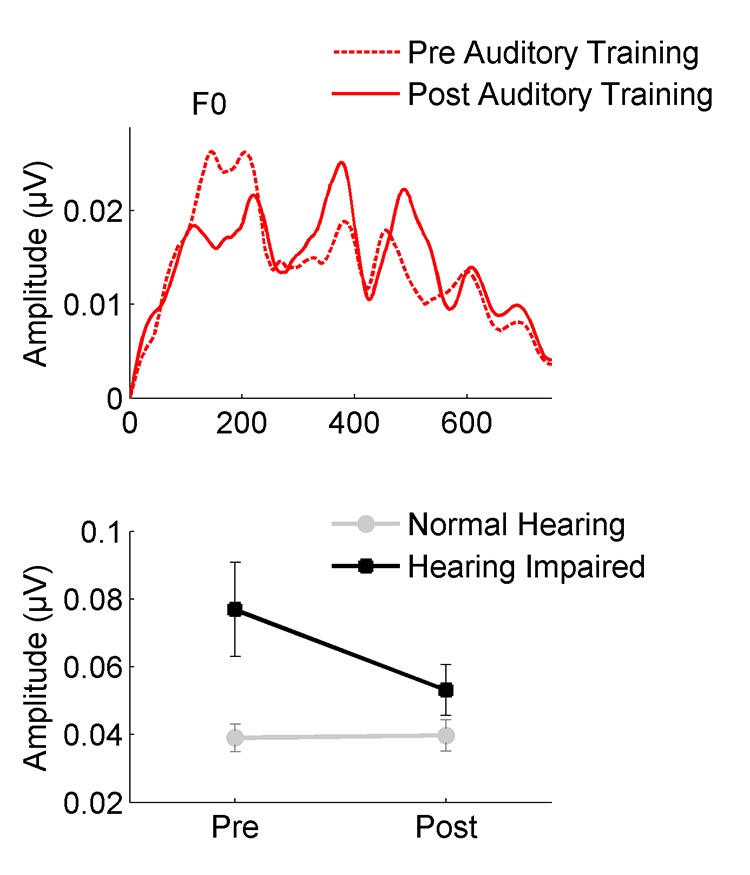
Figure 3. Auditory training reduces neural response amplitude to the speech envelope (F0) in participants with hearing loss. Exaggerated amplitudes noted at the pre-training session are not observed in the post-training session. (Adapted with permission from Anderson et al.5 with permission from Frontiers).
It may also be possible to reverse changes in neural excitability induced by hearing loss through the use of hearing aids (HAs). Karawani et al.7 recruited older adults with mild-to-moderate ARHL who had never worn amplification and fit them with Widex Dream 440 receiver-in-the-canal HAx. FFRs were recorded in both aided and unaided conditions. Half of the participants wore the hearing aids for six months and the other half did not. Pre- and post-FFRs showed that the fundamental frequency was significantly lower in the HA users after six months, whereas no change was observed in the non-HA users (Figure 4).
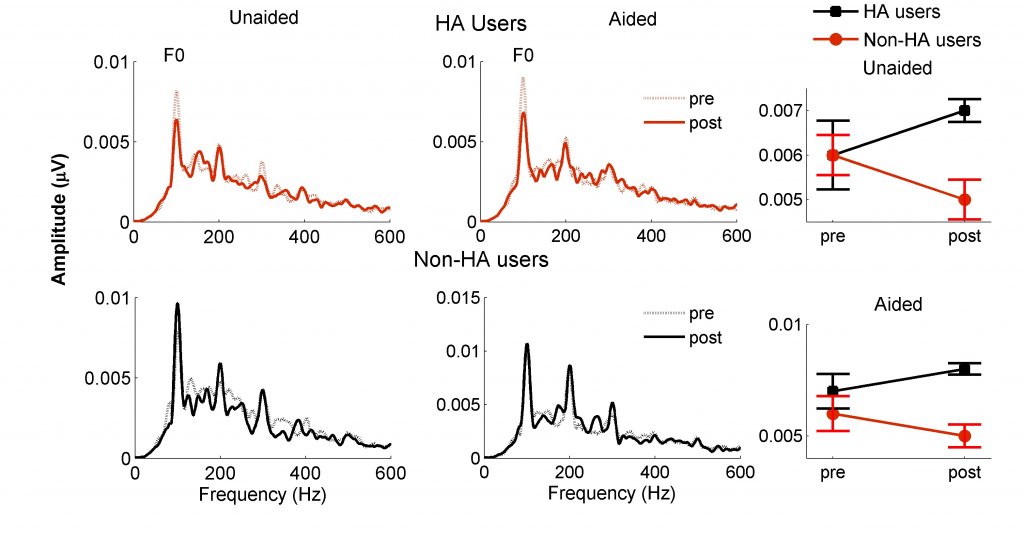
Figure 4. Six months of hearing aid use reduces the amplitude of the fundamental frequency (F0). Spectral amplitudes are lower at post-test in hearing aid (HA) users (red dashed line) compared to amplitudes at pre-test (red solid line). Similar changes were not observed in the non-HA users between pre-test (black dashed line) and post-test (black solid line). These differences are apparent in both aided and unaided recordings. (Adapted with permission from Karawani et al.7 with permission from Clinical Neurophysiology).
In conclusion, when encountering patients who struggle with the loudness of speech and other environmental sounds, we can counsel them about the changes that occur when the auditory system is deprived of sound. Fortunately, these changes may reverse over time when the patient begins to re-experience a normal sound environment, restoring the ability to enjoy listening to everyday sounds.
References
- Anderson S, Gordon-Salant S, and Dubno JR. Hearing and aging effects on speech understanding: Challenges and solutions. Acoust Today 2019;14(4):10–18.
- Guinan JJJ. Olivocochlear efferents: Anatomy, physiology, function, and the measurement of efferent effects in humans. Ear Hear 2006;27(6):589–607.
- Skoe E. and Kraus N. Auditory brain stem response to complex sounds: A tutorial. Ear Hear 2010;31(3):302–24.
- Coffey EBJ, Musacchia G, and Zatorre RJ. Cortical correlates of the auditory frequency-following and onset responses: EEG and fMRI evidence. J Neurosci 2017;37(4):830–38.
- Anderson S, et al. Training changes processing of speech cues in older adults with hearing loss. Frontiers Syst Neurosci 2013;7(97):1–9.
- Presacco A, Simon JZ, and Anderson S. Speech-in-noise representation in the aging midbrain and cortex: Effects of hearing loss. PLoS One 2019;14(3):e0213899.
- Karawani H, Jenkins KA, and Anderson S. Neural and behavioral changes after the use of hearing aids. Clin Neurophysiol 2018;129(6):1254–67.Samira Anderson looks at counselling patients on understanding the mechanisms involved in adjusting to increased loudness.